Daniel Carbonell, TRI-HP Project Coordinator and Technical Coordinator of BEST-STORAGE, details efficient solutions for heating and cooling using natural refrigerant heat pumps and high-energy density storage solutions.
Solar-ice slurry concept for efficient building heating supply
Using the energy from the Sun to supply large shares of heating demands in residential applications is a meaningful option for many climates, yet not easy to achieve while being cost competitive. The TRI-HP project shows how building heating demands can be efficiently supplied with solar energy, using what is known as solar-ice, in a cost-efficient way. This system concept combines solar thermal collectors, heat pumps and ice storage to supply the energy needs in heating-dominated regions with enough solar irradiation, e.g., Central Europe. The ice slurry produced in the winter and spring seasons can be used for free-cooling as an add-on feature in climates with low cooling demand.
Solar-ice systems use solar thermal collectors as the only heat source for the heat pump, which can additionally be powered by photovoltaics (PV). Solar thermal collectors are also used to supply heating and domestic hot water demands directly. As long as the Sun is shining, or the ambient temperature is not too low, solar collectors act as a direct heat source for the heat pump. During cold nights or days with low solar irradiation, ice storage is used as a heat source. The ice storage acts as a low-temperature solar seasonal storage (charged in summer and discharged in winter) with a very large energy density storage in the range of 80 kWh/m3. A conceptual scheme of the solar ice system can be seen in Fig. 1.
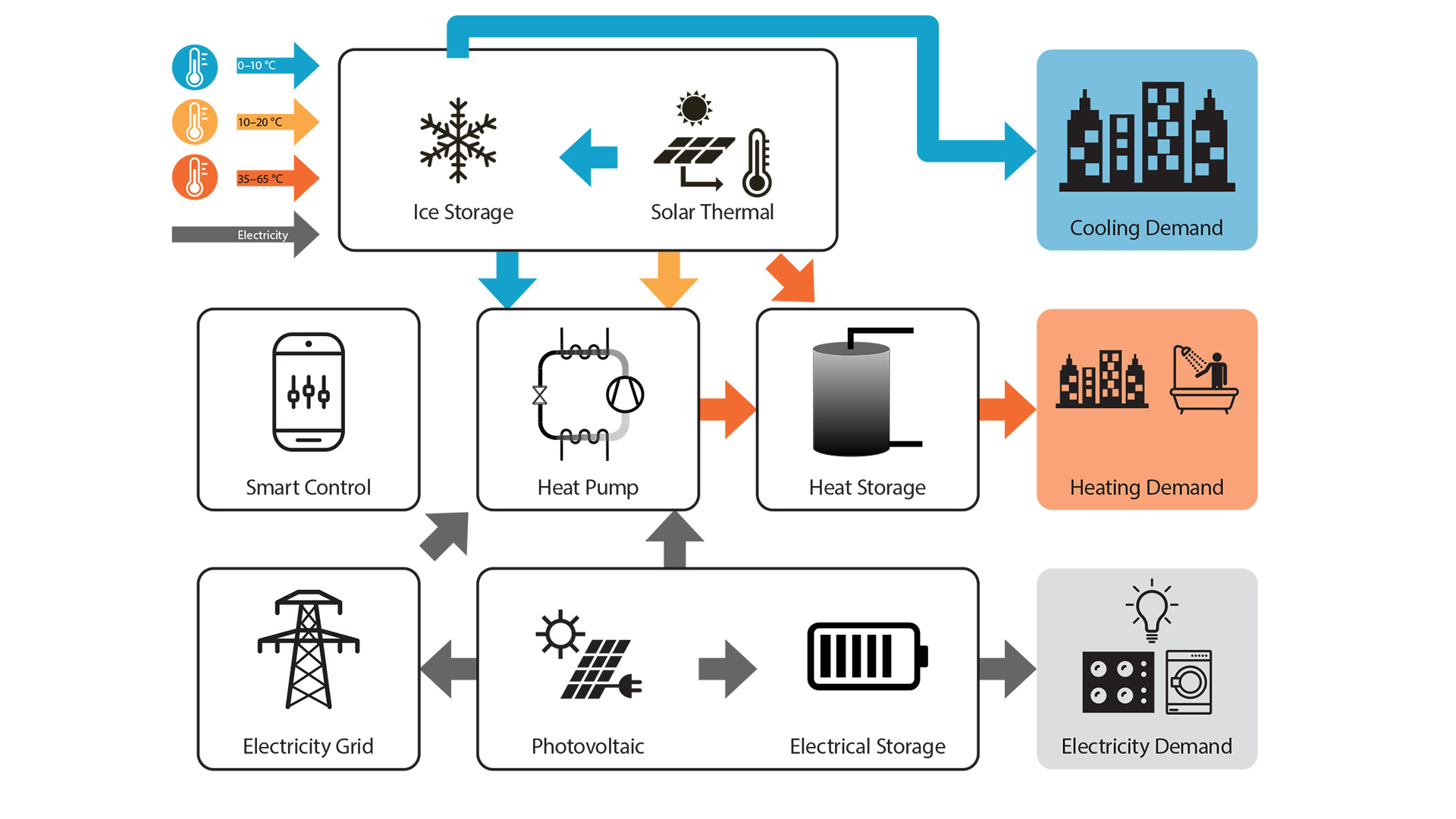
Solar-ice slurry concept for space heating and domestic hot water supply with free-cooling as an add-on feature
The solar-ice slurry system can be compared to ground source heat pumps (GSHP) with the benefits of not having to drill boreholes and thus not being restricted by water protection laws. Moreover, there is no need to regenerate the ground as in the case of boreholes, even if the storage is buried in the ground, since it regenerates on a yearly basis by solar energy.
The main innovation proposed by TRI-HP is the development of the ice slurry concept with the supercooling method, which eliminates the heat exchangers inside the ice storage, reducing the system installation cost by 10%. Moreover, the heat transfer surface (supercooler) is always free of ice and has a higher efficiency compared to conventional ice-on-coil systems. With this innovation, solar-ice slurry systems are expected to have a similar cost to GSHP for the same system efficiency without needing to drill boreholes or regenerate in future scenarios.
Ice slurry supercooling production concept
One of the main technological barriers for ice slurry systems using the supercooling method is developing heat exchangers that allow operating with water temperatures below 0°C without freezing. Within the TRI-HP project, we have developed durable icephobic coatings that work in turbulent water flows, allowing them to suppress ice formation for the necessary working conditions. Once water is supercooled in a stable form, it is pumped to an ice crystalliser, where the ice slurry is formed to be then stored in the ice slurry vessel. The supercooling degree is defined as the difference between the actual freezing temperature and the melting temperature, which is 0°C in the case of water.
The tested TRI-HP supercoolers based on brazed heat exchangers are extremely compact and reach supercooling degrees up to 4°C, which is well beyond the 2°C achieved by state-of-the-art Japanese technologies using less compact heat exchangers. The average supercooling temperature was evaluated for seven freezing cycles for different icephobic coatings, which are marked as dots in Fig. 2.
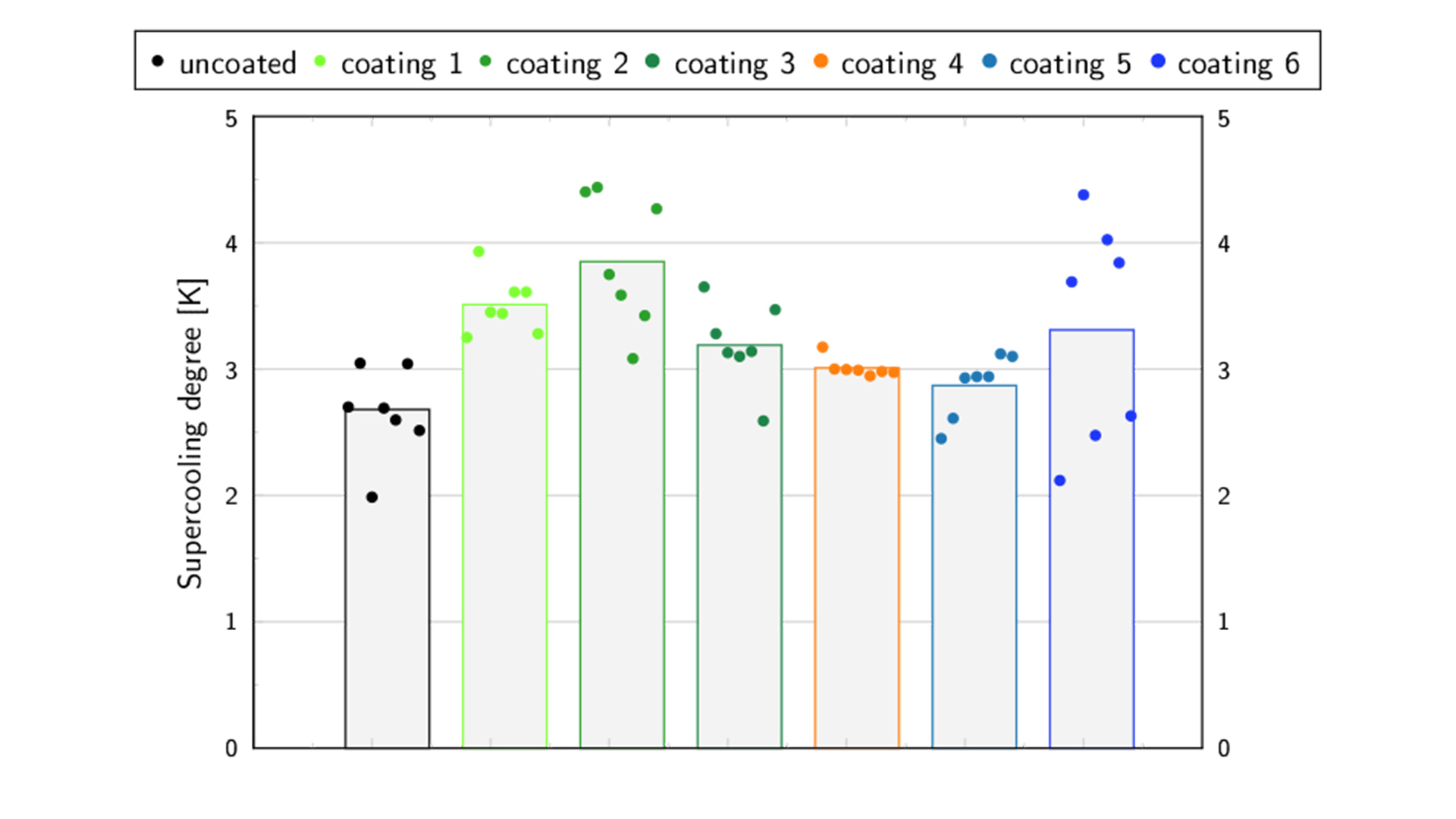
New ice slurry heat pumps with natural refrigerants
The use of synthetic refrigerants started around 1930. Since then, they have been regulated due to the destruction of the Earth’s protective ozone layer, high Global Warming Potential (GWP) and impact on human health. This has led to the development of three generations of synthetic refrigerants. The last generation, based on HFO, decomposes in the atmosphere, generating trifluoroacetic acid and forming trifluoroacetate, which can contaminate drinking water. Thus, the only long-lasting, sustainable solution for heat pumps is using natural and environmentally friendly refrigerants with low GWP, such as hydrocarbons, water, ammonia, and carbon dioxide (CO2). In this context, new heat pumps with natural refrigerants (propane and CO2) were developed and tested in the context of the TRI-HP project.
Within the TRI-HP project, the integration of supercoolers in residential heat pumps for heating applications has been successfully implemented. We have designed and manufactured two prototypes with capacities of around 10 kW of thermal power, one using propane and the second one using a transcritical CO2 cycle.
Results for the coefficient of performance (COP) of the two heat pumps are shown in Fig. 3. Data points at 0°C water inlet temperature in the evaporator show situations in which water was supercooled without any freezing, thanks to the icephobic coatings.
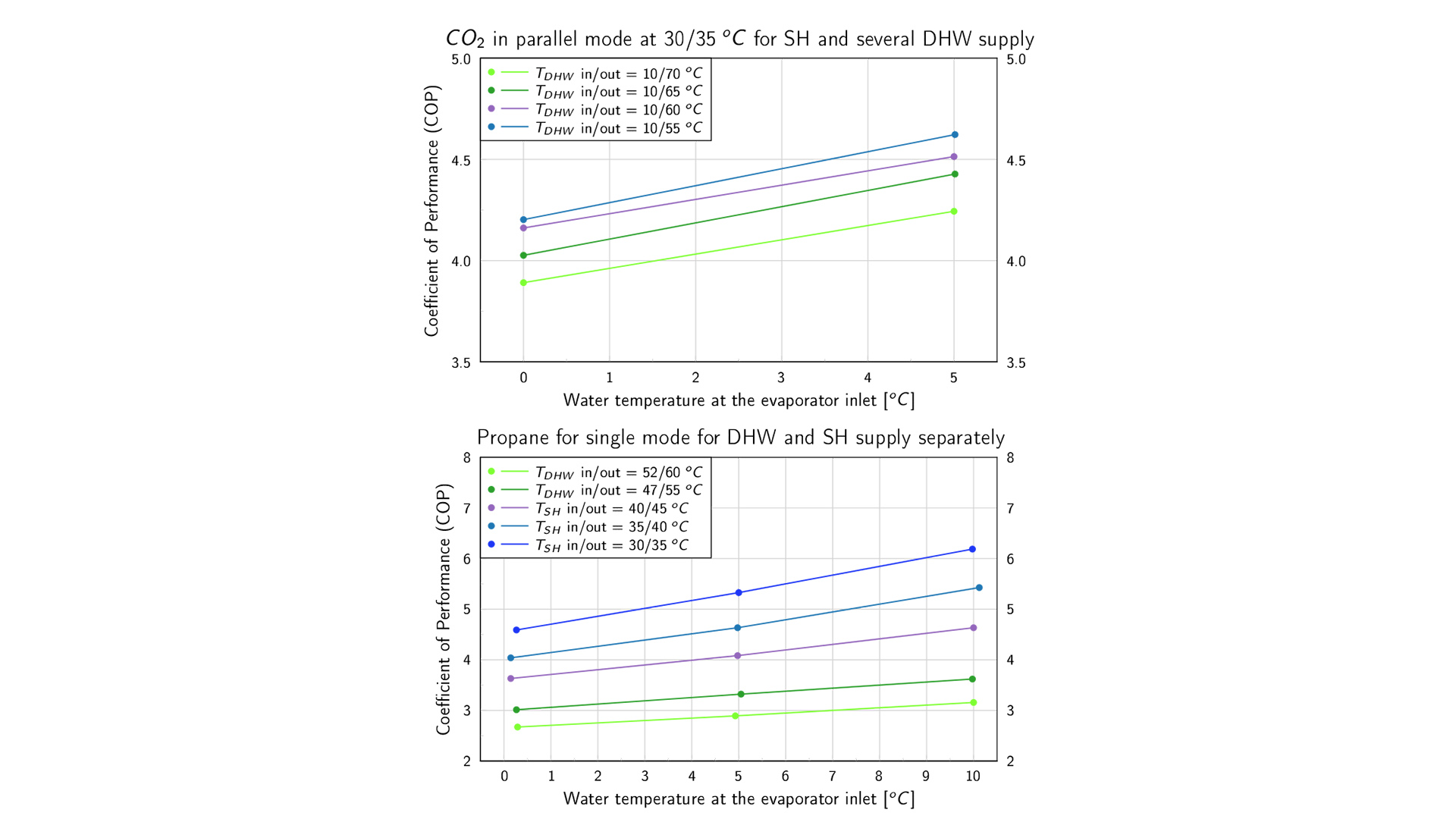
Besides the supercoolers, the CO2 heat pump had an additional innovation. The heat pump has a tri-partite gas cooler using three heat exchangers for the efficient supply, simultaneously or not, of space heating (SH) and domestic hot water (DHW) demands. In Fig. 3 (left, top), the Coefficient of Performance (COP) of the CO2 heat pump for simultaneous production of SH and DHW as a function of several DWH temperatures are shown. For these results, the SH demands are provided at 30/35°C for return/flow conditions from/to the radiant floor. The COP range from 4 to 4.5 in most cases while providing high temperatures for DHW demands. The heat pump is even able to supply DHW temperatures up to 70°C with a COP above 4.
The propane heat pump can operate either to provide SH or DHW demands separately. The COP for the provision of SH demands presented in Fig. 3 (left, bottom) is in the range of 4.5 to 6 for new buildings (supply at 35°C) and between 3.5 to 5.5 for refurbished ones (supply at 45°C and 40°C). The COP reduces to around 2.8 to 3.7 to supply DHW demands at 55°C and 60°C.
With these results, one can conclude that the CO2 heat pump is a much better option for new buildings with a large share of DHW demands. On the other hand, propane is a better candidate to provide a large share of SH demands.
Accelerated system test with hardware-in-the-loop
The validation of the solar-ice slurry systems was carried out using the accelerated concise cycle test (CCT), where the whole year is represented by seven days, each representing a specific period of the year. The CCT is based on a hardware-in-the-loop approach where the systems are operated autonomously in a relevant laboratory environment as if they were installed in a real building. The components developed in the project, as well as those necessary to create realistic boundary conditions, were physically installed in the laboratory, such as the natural refrigerants’ heat pumps explained above, the DHW and SH storage tanks, all hydraulic elements and connections between these elements as well as the overall control of the system that allows operating the system autonomously for several days in a row. The electrical battery and the inverter were also installed physically. The rest of the system, including the response of the solar thermal collectors, PV, ice crystalliser and ice slurry storage, were simulated and emulated. The building demands, including the response of the heating distribution system (radiant floor), DHW tapping profiles, and household electricity profiles, were also simulated/emulated to create realistic boundary conditions for the system operation. The installed and tested components in the laboratory were sized relative to the 10 kW heating power of the heat pump, and simulations were used to scale the system to higher multi-family building demands.
The ice slurry propane system targets a renovated multi-family building with a specific SH demand of 105 kWh per heated surface area and year in the city of Zurich. The system tested experimentally achieved an annual system performance factor (SPF), including the electricity consumption of the heat pump compressor and all circulation pumps, up to 4.8, which is significantly higher than typical SPF achieved by ground source heat pumps in this climate, which typical values are in the order of 4.
The ice slurry CO2 system targets a new multi-family building with specific SH demands of 30 kWh per heated surface area and year in the city of Zurich. This system was tested for 14 consecutive days, successfully reaching an annual SPF above four for a new building with high shares of DHW demands (60% of the SH demand including recirculation losses) at high temperatures. Fig. 4 shows the outlet temperature of the CO2 heat pump to the DHW and SH supply and the outlet of the evaporator (supercooler) during two winter days, followed by a spring day. For all the operating hours of almost two consecutive days, water was constantly supercooled while the heat pump was in operation (the ice storage was freezing), and not a single unwanted freezing was observed. The generation of ice out of the supercooling water and its storage in an ice slurry tank was simulated and emulated in this test. These results show the validation of the system in realistic dynamic boundary conditions in which the supercooling approach using a compact braze heat exchanger with icephobic coatings worked in a stable form. For a scale-up system, an ice storage of 56m3 and a collector area of 76m2 are necessary to cover the 51 MWh of total heat demand.
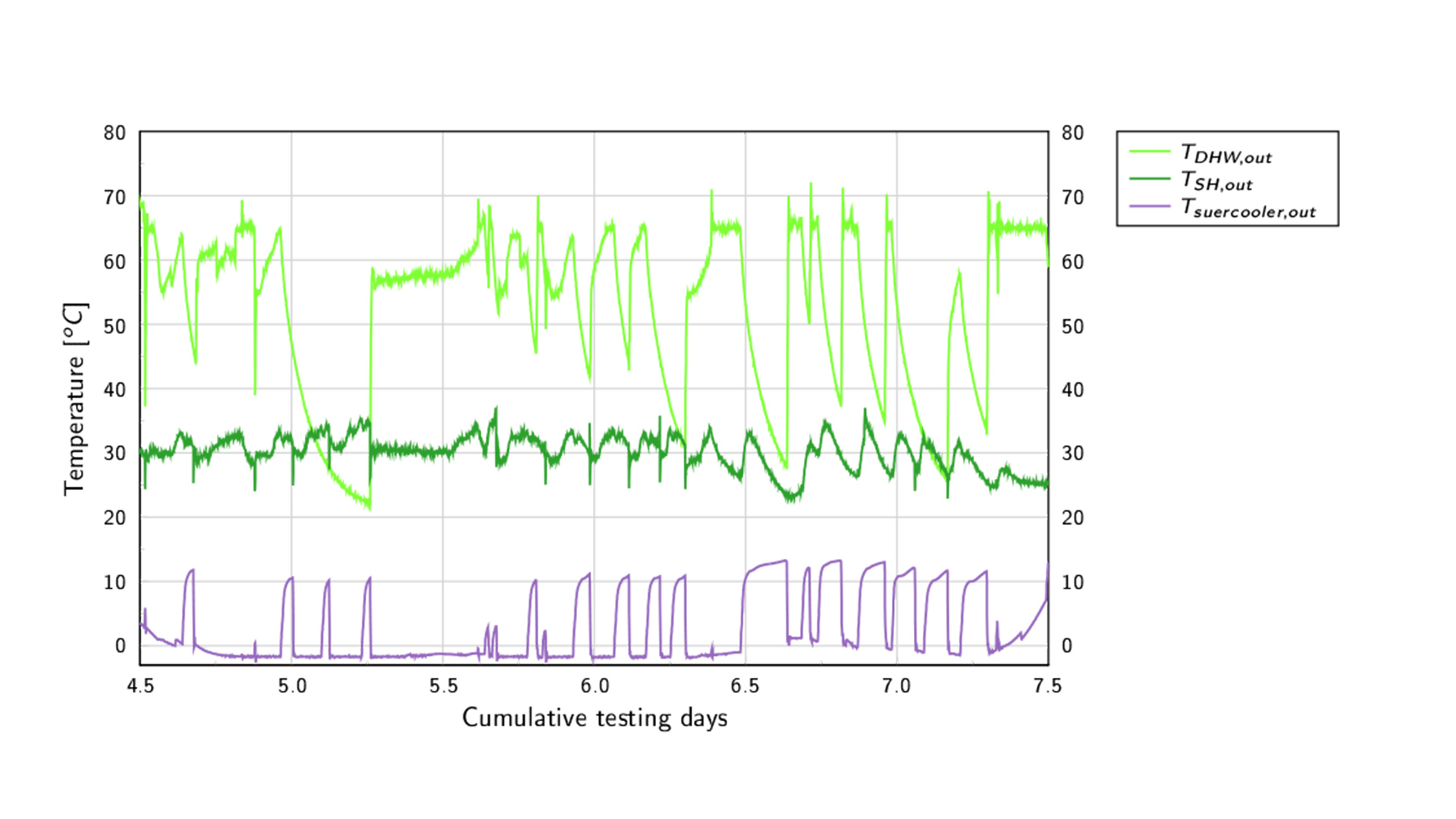
For the first time worldwide, a stable operation of a supercooling slurry heat pump system for residential heating applications has been validated in the laboratory, providing a significant technological step forward. Moreover, it was possible to use very compact braze heat exchangers that are commonly used in state-of-the-art residential heat pumps as supercoolers. The next step will be to test the complete system with a real ice crystalliser and ice slurry tank, which were not developed within the TRI-HP project and, therefore, not tested physically in this project.
High energy density storage for peak load cooling The ice slurry technology using the supercooling method developed in the TRI-HP project for an efficient heat source for heat pumps in residential heating applications can also be used for cooling applications. In fact, this is probably the highest market potential for this technology worldwide. The high energy density stored in ice can be used to cover the peak cooling loads for a few hours on a day, shifting electricity consumption to times when electricity is cheap or when PV electricity is available. However, the chiller efficiency drops significantly when operated below 0°C to produce ice compared to water storage, where energy can be stored much closer to the cooling demand-supply. To deal with this problem, the follow-up BEST-STORAGE project will develop a Phase Change Material (PCM) slurry system able to store energy at around 6 to 12°C with an energy density in the range of 35 kWh/m3, which is six times larger than water storage with a temperature difference of 6°C. Moreover, the chiller efficiency will increase by at least 20% compared to the ice storage due to the higher melting temperature of the PCM. The BEST-STORAGE project started in January 2023 and will demonstrate the PCM slurry technology for shifting peak cooling loads in real applications during 2026.
High energy density solutions for seasonal energy storage
In the solar-ice concept explained above, an ice slurry storage acts as a low-temperature seasonal storage being charged by solar energy in summer to be discharged mostly in winter as a heat source for the heat pump. Nevertheless, the heat pump has to use electricity which availability might be short in the coldest winter periods in fossil-free future energy scenarios. In general, large amounts of energy are needed for the heat supply of buildings in cold winter months, when solar energy is scarce, and renewable sources cannot cover the full demand. Thus, seasonal storage solutions able to provide heating demands will be necessary to decarbonise the energy supply systems fully. Within the BEST-STORAGE project, a high energy density storage based on a thermo-chemical technology will be further developed and demonstrated in the field with supply heat capacities up to 8 kW. The storage concept works as a thermally driven sorption heat pump based on aqueous sodium hydroxide (NaOH), an abundant and very cheap material. With this technology, excess energy from intermittent renewable sources such as PV, solar thermal or wind is stored without any losses to be used later during the coldest winter periods.
TRI-HP Consortium
TRI-HP involves 11 partners from seven different European countries (Belgium, Denmark, Germany, Norway, Spain, Sweden and Switzerland): seven R&D partners (SPF-OST, TECNALIA, IREC, ISOE, NTNU, DTI, UASKA), three SMEs (HEIM, ILAG), a large industry partner (ALFA LAVAL) and an NGO (REHVA). The project is coordinated by SPF-OST.
BEST-STORAGE Consortium
BEST-STORAGE involves 12 partners from seven different European countries (Belgium, Estonia, Germany, Greece, Netherlands, Spain and Switzerland): three R&D partners (CERTH, TECNALIA, TEKNIKER), three universities (SPF-OST, SUPSI, TUB), a large industry partner (GIROA/VEOLIA), three SMEs (AVA, NEWTON, SOLINTEL) and two non-profit associations (EHPA, TREA). The administrative coordination is carried out by SOLINTEL, and the technical coordination is led by SPF-OST.
TRI-HP project has received funding from the European Union’s Horizon 2020 under grant agreement No. 814888. BEST-STORAGE project has received funding from the European Union’s Horizon Europe under grant agreement No. 101096516.
Please note, this article will also appear in the thirteenth edition of our quarterly publication.
Thanks for sharing, air source heat pumps are a popular and effective way to heat and cool a home because they use less energy, are flexible, and are easy to install and keep up. But when choosing an ASHP system for a certain application, it’s important to think about things like the climate, the size of the building, and the budget.