Massive stars in extreme evolutionary stages have been studied to reveal their pulsation and mass loss properties, indispensable for the understanding of stellar and galactic evolution.
One of the most important, long-standing questions in astrophysics is: How do massive stars evolve from their birth up to supernova explosion?
Massive stars are powerful cosmic engines. Throughout their entire lifetime, they enrich their environment with huge amounts of energy and chemically processed material. The energy released from massive stars is so powerful that it can trigger the formation of new generations of stars and planets. The chemical elements produced inside stars and released to their environment via winds and eruptions are the building blocks of all life as we know it on Earth.
Despite the importance of massive stars for cosmic evolution, their aging process from the cradle to their death as spectacular supernova explosions still faces many uncertainties.
The EU-funded project POEMS aimed to remove some of them, in particular in relation to precise mass-loss predictions in various late evolutionary stages that were so far hampering reliable predictions for the evolution and fate of massive stars.
Creating the POEMS project
For the implementation of the project, we established a multidisciplinary, international network of researchers from Europe, Asia, and South America with expertise and background in both theory and observations and in a variety of disciplines such as astronomy and astrophysics, fluid dynamics, nonlinear physics, molecular physics, astrochemistry, statistics and applied mathematics.
The research focused on diverse aspects:
1. We investigated new ways to determine exact hydrodynamical solutions that prescribe the winds and mass loss of stars in extreme evolutionary phases
2. We studied the impact of pulsation and other instabilities on mass ejections and outbursts, and examined the structure and dynamics of the ejected matter along with the formation of circumstellar rings and disks
3. We elaborated how the wind material interacts with the interstellar medium.
New mass-loss prescriptions
Hot, massive stars lose mass via line-driven winds. Our analysis reveals new, exact hydrodynamical solutions of the wind velocity distribution, which is of utmost importance for predicting the radial density distribution in the winds and, hence, the total mass loss of a star in a specific evolutionary state.
We are implementing these new solutions into state-of-the-art numerical codes (FASTWIND, CMFGEN). With these, we compute self-consistent wind solutions together with mass-loss rates and emerging spectra of stars spreading over a large initial mass range.
These new mass-loss rates are significantly lower than previous values. Consequently, evolutionary tracks computed with these new mass-loss recipes predict that stars will remain massive and evolve at higher luminosity, leading to a significantly larger amount of stellar black holes.
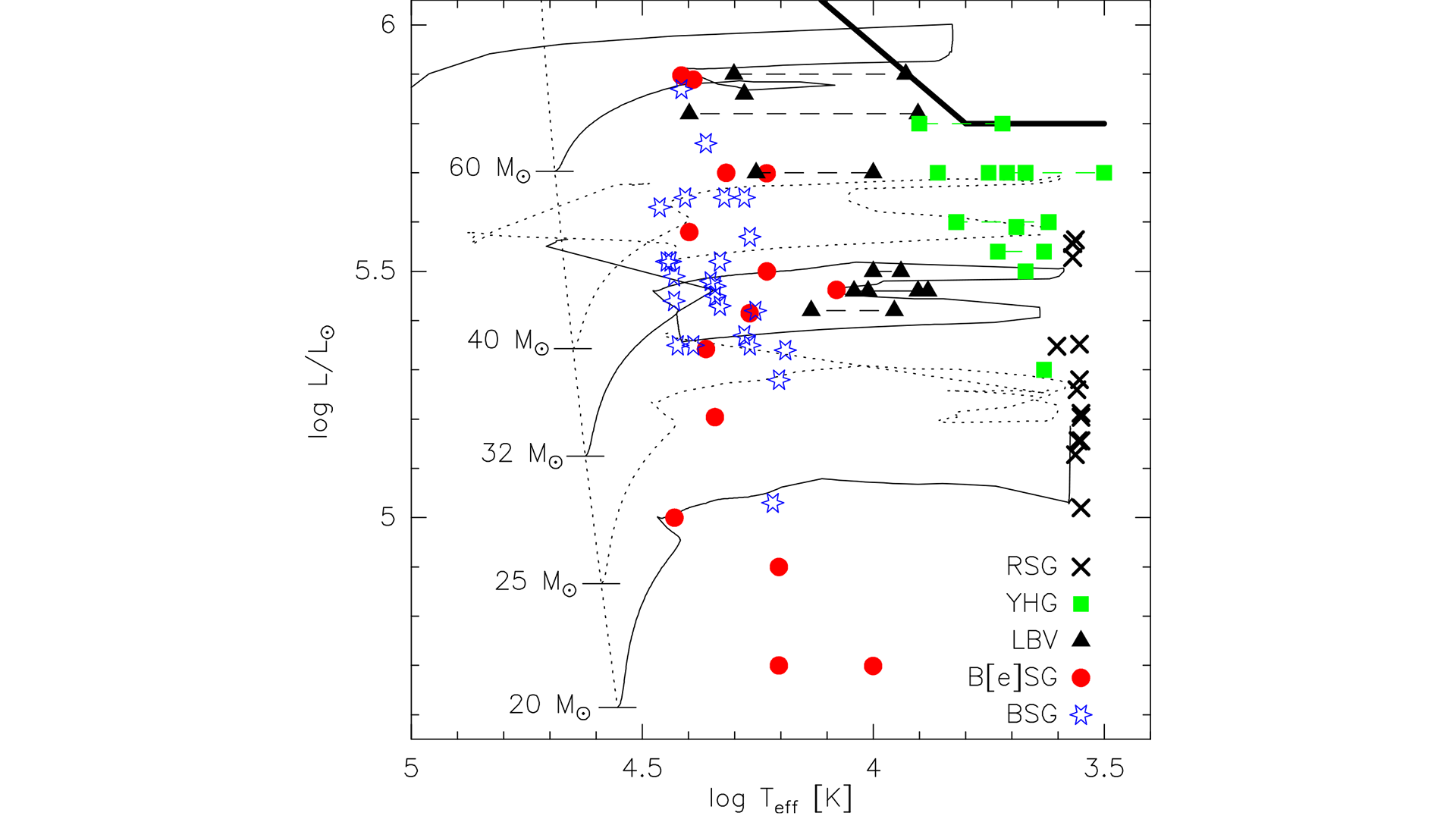
Pulsations and hydrodynamical instabilities inducing mass ejections
In addition to their line-driven winds, massive stars can also lose mass during certain evolutionary phases through mechanical processes such as pulsation-induced mass loss and eruptions. For pulsation instabilities to be effective (so-called strange-mode instabilities), the stars should have a high ratio of their luminosity over mass (>10⁴ in solar units), which is easily achieved by stars in their late evolutionary stages, that is, after having lost a significant fraction of their initial mass through winds.
We focused on stars in the initial mass range of 20-40 solar masses. Evolutionary calculations predict that these objects pass through a red-supergiant phase in which they shed large amounts of material and then pass through a yellow hypergiant and a follow-up blue supergiant phase (Fig. 1) before exploding as supernovae. The envelopes of these highly inflated objects are very prone to even the smallest perturbations.
Our analyses of such perturbations reveal that pulsational as well as hydrodynamical instabilities can occur with velocity amplitudes exceeding the escape speed and lifting surface material into the star’s environment.
In this way, mechanical mass loss (or even an outburst) is produced that tops up the mass loss from the stellar wind. Our detection of clear correlations between pulsation periods and phases of enhanced mass loss in spectroscopic time-series observations and of signals in photometric light curves with periods that are in the same order as our theoretically predicted ones underline the reliability of our investigations.
Regular pulsations are also excited in a second population of blue supergiant stars. These are objects that have just left the main sequence. Compared to their more evolved post-red supergiant counterparts, these stars still have significantly higher mass by otherwise the same temperature and luminosity.
We have investigated the pulsation properties of a sample of blue supergiants to classify them according to their (pre- versus post-red supergiant) evolutionary phase. The results will allow us to study the deep interior of these stars, which is not accessible by any other means, along with more reliable values of their masses.
Structure and dynamics of circumstellar matter
The matter that was released from the stellar surface by either winds or mass ejections can accumulate in the vicinity of the star, creating splendid structures such as disks, rings, or shells. A specific group of evolved massive stars with circumstellar rings are the B[e] supergiants. Their environments are so stable that chemical processes can take place, leading to the formation of molecules and dust.
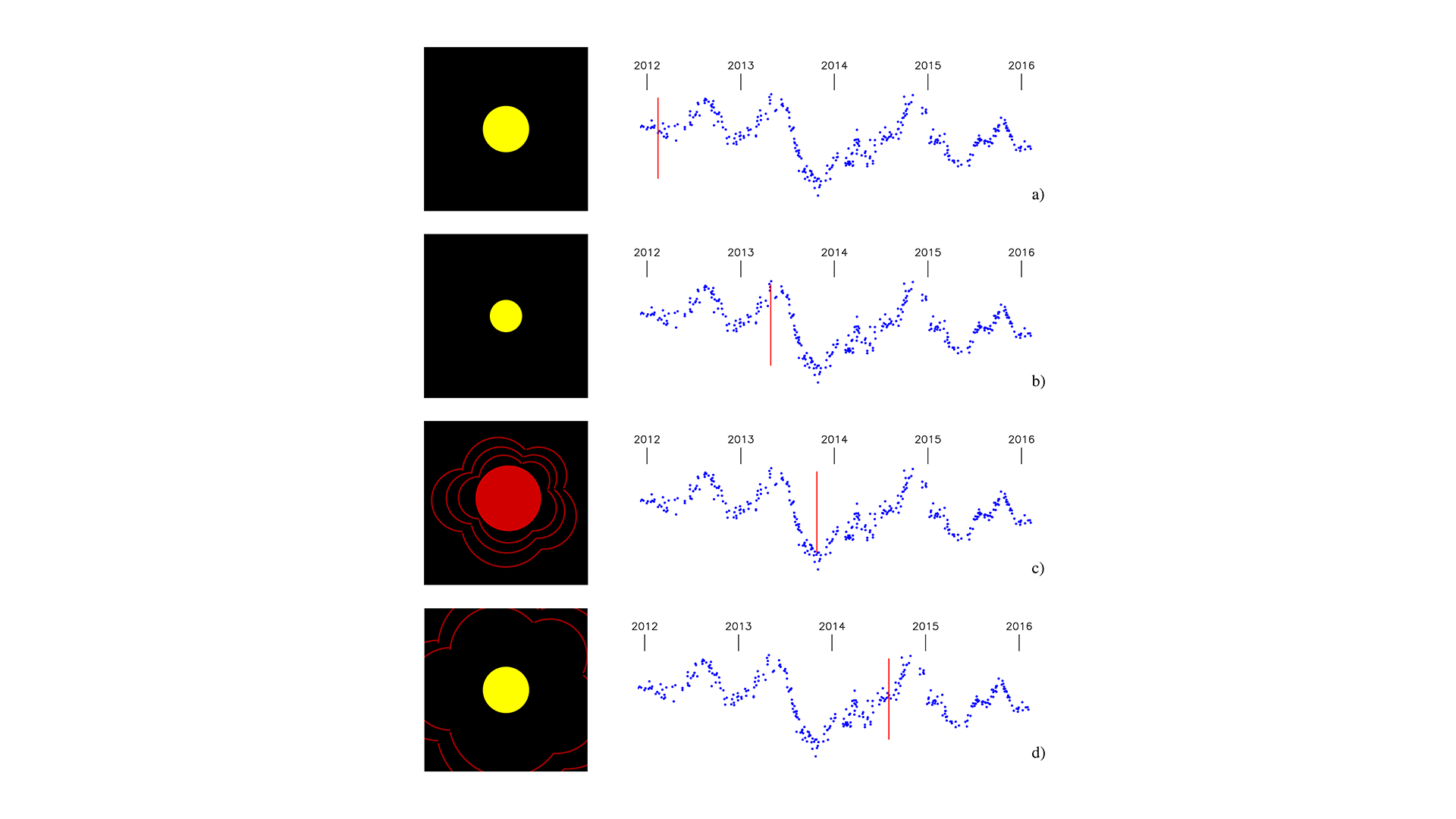
The rings around most objects were found to revolve around the stars on Keplerian orbits, and we detected the emission from various molecular species using modern observing facilities at the European Southern Observatory (ESO) and GEMINI Observatory. These discoveries help characterise and understand the ongoing chemistry.
The outbursts of yellow hypergiants can occur on time scales that range from decades to centuries. They imprint their signatures on photometric light curves (Fig.2). These outbursts can also yield observable structures around the central star traceable by either molecular or dust emission. We have observed many yellow hypergiants and discovered multiple dust shells, circumstellar gas disks composed of carbon monoxide and hot water vapour, and bipolar outflows.
A subgroup of massive main-sequence stars displays phases of disk formation and dissipation. These are the classical Be stars. It is generally believed that disk formation is related to critical stellar rotation, and our studies reveal that the spinning-up of the stars to critical rotation could be caused by mass transfer in very close binary systems.
However, we have also found many Be stars that generate disks without rotating critically, posing new challenges to our understanding of their disk formation process.
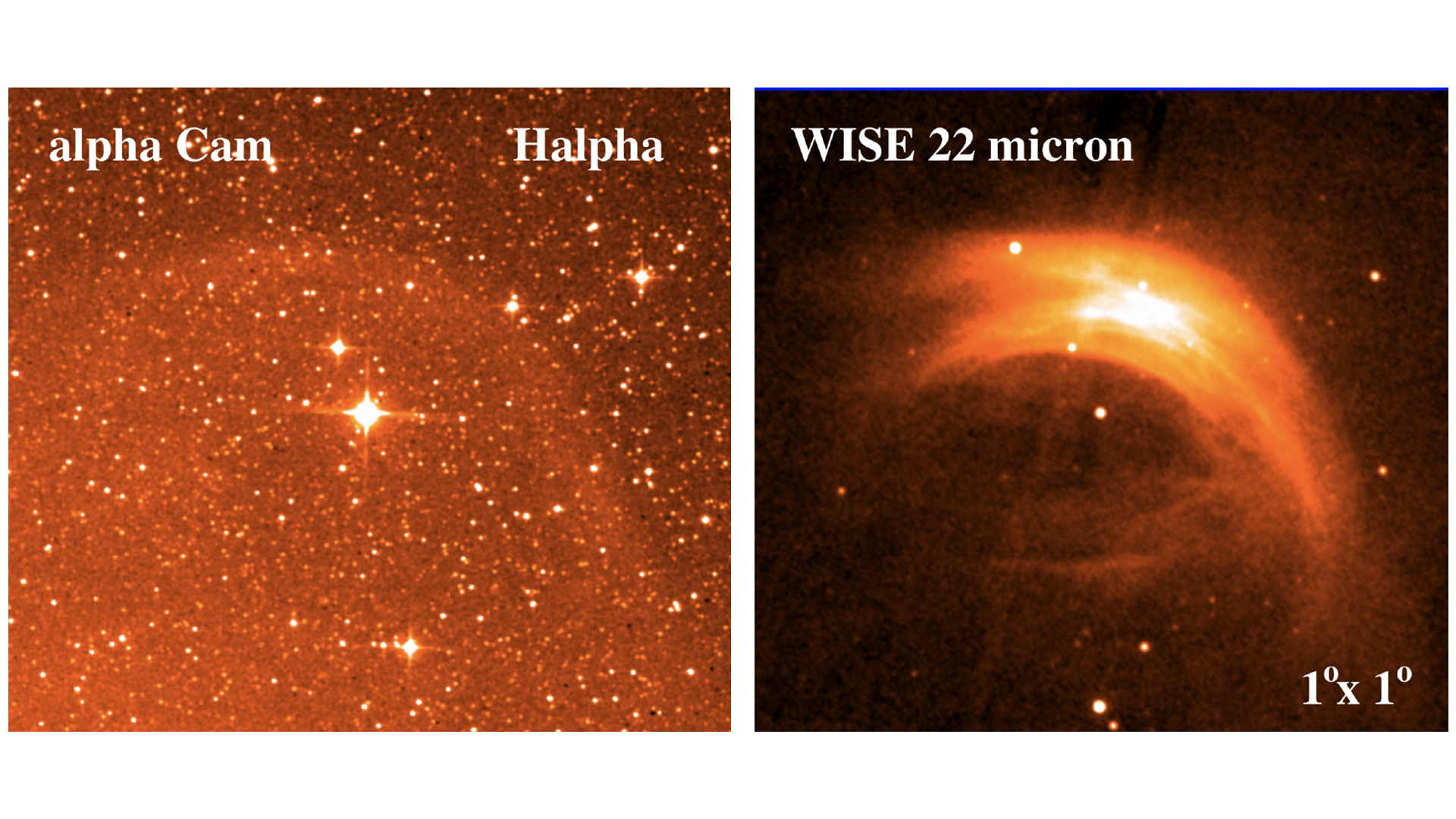
Interaction of the wind with the interstellar medium
Since stars travel through the local interstellar medium, the wind material of massive stars impinges on the interstellar medium, creating observable bubble structures known as astrospheres. For supersonic flows, the interstellar material is hereby compressed and heated, producing bright dust emission along the bow shocks detectable at infrared wavelengths. Due to the high density of the wind material in certain evolutionary stages, we speculated that the shocked wind material should also cause observable emissions.
Therefore, we carried out an imaging survey at optical wavelengths centered on the well-established wind emission line Hα and detected a significant fraction of these bow shocks (Fig. 3). Further analysis will provide insight into the parameters of the stellar and interstellar plasma flows and help deepen our understanding of the formation of bow-shocks.
Besides scientific research results, our project also provides databases that can be exploited by the community. To date, we have released a large grid of synthetic models (ISOSCELES) and a database with molecular spectra. Furthermore, we are releasing the observations taken with various national facilities throughout the project period.
It is noteworthy that the research supported by this project is at the forefront of science in several research areas, such as stellar wind modelling and observing, and modelling the environment of evolved massive stars. In addition, our team developed cutting-edge models, software, and tools that can be further exploited in various disciplines.
The team is also extremely efficient in getting assigned observing time with most modern observing facilities to collect the best possible data (often the first of its kind), processing and analysing them. We expect that the final results of POEMS will advance our understanding of physical phenomena, foster innovation, and drive sustainable developments that serve both the scientific community as well as society.
Acknowledgement
This project has received funding from the European Union’s Framework Programme for Research and Innovation Horizon 2020 (2014-2020) under the Marie Skłodowska-Curie Grant Agreement No. 823734 (POEMS).
Please note, this article will also appear in the 18th edition of our quarterly publication.