Alexander Fairtlough, Chairman of the Board, and Pedro Mayorga, CEO of EnerOcean S.L., detail how achieving advanced technology certification from classification bodies can help speed up global deployment of cost-effective offshore floating wind.
The article gives a snapshot of offshore and floating wind status in March 2024 and highlights current challenges in delivering the pipeline of projects required by policy priorities.
Especially for floating wind, the years to 2030 are crucial because sufficient capacity must be deployed to demonstrate progress towards reaching cost targets. Yet, investors hesitate to go entirely into floating as long as costs are high.
Enerocean’s W2Power solution, which offers better cost-reduction prospects by its inherent design advantages than floating technologies deployed at full scale so far, is advancing towards markets.
W2Power shows how achieving advanced technology certification from classification bodies can help to prove a technology, reduce risk, mature innovative solutions, and speed up global deployment of cost-effective floating wind.
Offshore wind: A snapshot
Offshore wind achieved a historic cost reduction in the ten years to 2023. In Northwest Europe, the average levelized cost of electricity (LCoE) per megawatt hour from offshore wind was €181 in 2012 but dropped to a range between €50 and €71 in 2022, corresponding to an almost 60% reduction1.
In 2023, the EU installed 17 gigawatts (GW) of new wind energy, slightly up in 2022 and a new single-year record2. Of the new capacity, 14 GW was onshore and 3 GW offshore.
In Dutch waters, a 1.5 GW wind farm was inaugurated using 139 200m rotor diameter turbines, each 11 MW. For now, it is the world’s largest offshore wind farm in operation – but it will be surpassed by the UK’s Dogger Bank, which will be 3.6 GW at total capacity (2026). Wind delivered on average 19% of all electricity produced in Europe in 2023. Nevertheless, the 17 GW built in 2023 is well below the 30 GW/year the EU needs to meet its 2030 climate and energy security targets.
As for offshore wind, Europe (EU and outside) and China each had 31 GW in operation by mid-2023; China had installed the most capacity in the last three years (12.7 GW in 2021, 6.8 in 2022 and 6.7 in 2023). In Europe, the UK leads with 11.3 GW operational offshore at the end of 2023, followed by Germany at 8 GW and Denmark and Belgium at 2.3 GW each.
In China, the province of Jiangsu is in the lead with 12 GW, before Guangdong (8 GW), trailed by eastern coastal provinces Fujian, Zhejiang and Shandong, each approaching 4 GW. In contrast, the shallow southern North Sea dominates European offshore wind; much of China’s offshore wind is in inter-tidal zones3.
Floating offshore wind
Floating wind is at the stage of deploying pre-commercial arrays. Five are operational in Scottish, Portuguese, Norwegian and French waters, with Provence Grand Large in the Mediterranean at the latest.
These small wind farms feature from three to eleven turbines. The first came on stream in 2017 and is illustrated in Fig. 1; the largest operational (March 2024) is Norway’s Hywind Tampen, with eleven 8-MW turbines supplying electricity to offshore oil & gas installations. The most powerful turbines, at 9.5 MW, are operating at Scotland’s Kincardine, with five floaters operational.
The five arrays are closely trailed by four technology developers testing large demonstrators of their currently proposed technologies, with turbines of 2-6 MW power, and by a pack of developers busy scaling up smaller prototypes to achieve this level offshore France, Japan, China, the US, Norway, and Spain. Both steel, concrete and combinations of these are used.
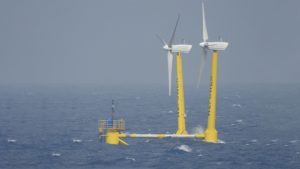
Fig. 2. The W2Power scaled prototype, undergoing tests in the open sea off Gran Canaria since 2019
In the five arrays, all three main classes of floating support are used: Spar buoys, semi-submersibles, and tension-leg platforms, and a barge-type design is represented as 2-and 3-MW demonstrators. The following contenders use these varieties, with Enerocean’s W2Power distinguished by featuring a pair of turbines on the same semisubmersible.
Looking ahead, bottom-fixed and floating offshore wind has established a huge pipeline of new projects under preparation, encouraged by governments setting ambitious targets of 100 GW for 2050, 2040 or even 2030. Some do more; in 2021, the Scotwind allocation round awarded acreage for 27.6 GW offshore wind in the windy seas off Scotland – 65% of it floating.
Today, many bottom-fixed projects are moving forward despite cancellations due to a mismatch between expected revenues and rising costs.
However, for floating, the gap between a healthy pipeline of more than 100 GW4 (some say more than 2005 and slowing near-term progress is growing. In 2020-21, most observers counted on 16 GW or more of floating to be installed by 2030, but recent studies range from 1 or 2 GW globally (Aegir Insights) to 7.5 GW (Douglas-Westwood).
By current estimates, few, if any, full-scale floating wind farms – with hundreds of turbines – are likely to be in service before 20306. It was clear from a mid-2022 interview study that, without any significant and early changes to the global framework of support and financing, developers plan to bring on stream-only floating arrays or small farms of a few hundred MW, at most, in the next five years7. The high cost of floating arrays built so far is making investors cautious.
While the floating pipeline remains strong and very well diversified across countries and continents, this growing mismatch between pipeline (>100 GW) and actual GW deployments (<10 GW) by 2030 raises a problem for floating: How to scale relatively immature technologies in a market where the volume stays low, whilst bottom-fixed is inaugurating more and more multi-GW farms? Could such an ‘embryonic’ industry (Aegir) be seriously delayed in delivering its great promise?
After all, floating is critical to bringing offshore wind to those regions that lack shallow seas – most countries.
All renewables that have successfully reached the market have needed volumes. Governments are used to provide incentives for new solutions until commercial investors are ready. A plan to license 4.5 GW of floating in the Celtic Sea in the UK will be awarded in 2024.
France, where two more 24-27 MW arrays are under construction in addition to one recently inaugurated, maintains its planned award in 2024 for two 250MW floating farms in the Mediterranean, with more than €4Bn in support already secured8. Official plans have these operational by 2031, and each can be extended to 750MW. Despite several postponements, Norway’s Utsira North floating wind tender (up to 1500MW) also remains on the cards.
Spain, Portugal, and Taiwan have plans for tenders of similar scale, if less advanced. So clearly, there are opportunities for projects to get funded and built for switch-on, if not by 2030, then between 2031 and 2035.
However, there may be other reasons, specific to floating, for its slow progress. For a start, value chains for floating wind differ from bottom-fixed. Only a part of the industrial construction base around shallow-sea areas suitable for bottom-fixed foundations – Northwestern Europe and the East and South China Seas – carry over into floating wind. Many deep dives into the cost reductions achieved in bottom-fixed offshore wind revealed that the lion’s share was due to the increasing size and power of wind turbines.
The industry achieved great reductions in CapEx per MW by using fewer turbines for a given power. In a nutshell, operations on fewer turbines reduced overall costs, even if each operation was more expensive due to turbine size and weight.
2023 saw records for wind turbine power. The two most powerful machines today are 16MW, commissioned and operated as prototypes in larger offshore wind farms off China.
The first deployed, Goldwind’s 252-16MW, beat the world record in 24-hour power output previously held by the V236-15MW of Vestas and before that, Siemens Gamesa’s SG14-222DD, both set during onshore testing at Denmark’s Østerild prototyping facility. MingYang’s also 16MW-rated machine has a 260m rotor. The same company leads in new machines with the MingYang 22MW, which will have a ‘31x-meter rotor and be commercially available for 2024/25 deployment.
Today, 15- to 18MW-rated turbines are becoming part of new planned offshore developments, but only on bottom-fixed foundations.
This is because, with floaters, different design criteria apply when scaling up to bigger turbines. One often overlooked aspect is that bigger, taller, and heavier turbines will increase the loads on floating support more than for the monopiles that carry most fixed offshore turbines. To keep a floater safe for a bigger turbine, it needs to be made larger and sturdier, with more construction steel (or concrete, which always includes steel rebars) in the structure, to keep its hydrodynamic stability. But this increases its weight, thus higher cost, also per MW.
Therefore, scaling up a floating wind system requires iterated, often complex design, verification and re-design loops and is a challenge. W2Power offers an advantage in that a pair of, e.g., 10MW turbines creates less of an overturning moment than one single 20MW turbine.
The large number of floating concepts in (often quite early) stages of development has also been said to pose a barrier to commercialisation9. Too much diversity can prevent supply chains from adapting their manufacturing and restrict turbine OEMs’ ability to adjust components for floating systems. The direction of technology development dictates port infrastructures, but they find it hard to plan in the absence of one leading technology.
In sum, this uncertainty limits banks’ ability to mitigate or reduce real or perceived technology risks. However, this argument is somewhat flawed since all the floating arrays built to date are very far from commercial; their LCoE and CapEx count in multiples of those for bottom-fixed wind farms. It would be wrong to pick the ‘most mature’ because they may be dead-end technologies regarding their ability to scale.
Only when the industry can settle on relatively mature technologies and have a favourable outlook for cost reduction by industrialisation will the floating wind sector benefit from the standardisation process enjoyed in other, more mature industries.
Classification and certification
The classification societies could be vital in bringing about many of the needed advances for floating. These non-governmental organisations establish and maintain technical standards for all floating structures.
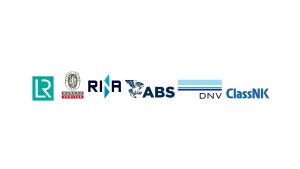
Classification societies certify that vessels’ construction complies with relevant standards and regularly survey in-service structures to ensure continuing compliance with the standards. Well known in the maritime sector, where insurers and investors rely on their services for all structures in use on the world’s seas, these have not had a prominent role in most forms of renewable energy, primarily terrestrial.
With offshore wind, and especially floating, this is changing.
Traditionally, the classification societies have been the ‘auditors’ of naval engineering. They ensured design, construction, and operations were conducted according to well-defined and documented regulations.
Today, they are playing an increasingly crucial role in developing and implementing floating offshore wind projects and technologies. They perform studies and services such as:
- Technical assessment of floating wind turbine designs, including structural integrity, fatigue, and load analysis. These assessments help to ensure their safety, reliability, and performance.
- Certification services for floating wind structures and components. This includes certification of design, production, installation, and O&M (operations and maintenance) processes.
- Risk management for Offshore Wind projects, already established for bottom-fixed, helping developers, investors, and operators to assess and manage risk. For floating, it also includes assessments of the units’ design, construction, installation, operation, and frequent environmental evaluations.
- Standards development includes new industry standards for floating offshore wind. The development of standards for the design, construction, operation and standards for offshore grid infrastructure is already established.
Most certification bodies also do advisory services, e.g., project management, environmental impact assessments, and regulatory compliance, and they perform R&D to improve their services.
Approval in Principle (AiP) versus Basic Design Approval (BDA)
The companies offer various levels of certification, but the distinctions between these are not always well understood in the renewable energy market. Here, we review two important ones for floating wind.
As mentioned, there are many proposed floating concepts, some of which are mere design ideas. The first step to maturity is an ‘Approval in Principle’, also known as a ‘Statement of Feasibility’.
Only a few have achieved full design approval from a classification society, such as the ‘Basic Design Approval’ (the names are those used by BV and DNV and may differ, but the content is essentially the same).
Approval in Principle (AiP) is a preliminary assessment of a design, indicating that it meets relevant rules and regulations set by the classification society. An AiP is a non-binding evaluation and does not guarantee future approval for construction. AiP is a valuable tool for offshore operators, designers, and builders, as it provides an early assessment of the feasibility and compliance of their design with the relevant standards, which are constantly evolving.
Basic Design Approval (BDA) is the final approval of a design and the authorisation to proceed with the construction of the asset. A BDA is only granted after a comprehensive design review, including detailed calculations, drawings, and specifications. The BDA is a binding evaluation and certifies that the design meets all the relevant safety, environmental, and performance requirements set by the classification society.
In short, the first step (AiP, SoF, or similar names used by other classification societies) provides an early evaluation of the design’s feasibility and compliance with the relevant standards. This can be obtained based on limited or initial design calculations and drawings. It is relatively cheap, so small companies and start-ups achieve them, often exaggerating their importance to tempt investors.
A design approval, however, represents the final verification of a design and implies an authorisation to proceed to large-scale construction. A BDA results from thousands of engineering hours, drawing on comprehensive and sophisticated simulations, model tank testing and often strong involvement by the wind turbine generator (WTG) supplier. If the technology certified is based on a well-proven WTG for offshore use and a well-known mooring design, the risk of surprises is further minimised.
A recent certification of this advanced type is that of Enerocean’s W2Power. As reported in the specialist press at the end of 202310, with scenes from the public awarding ceremony illustrated in Fig. 4, the W2Power technology is proceeding to a full-size demonstrator located in a commercial port.
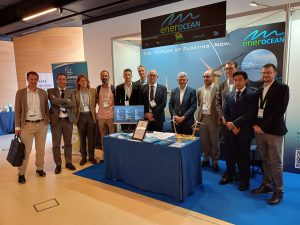
Fig. 4. Scenes from BDA certificate award ceremony with BV and Enerocean representatives
Having received the AiP from BV in 2021, the mature and now certified W2Power design offers the floating industry’s highest MW power per tonnage rating, a useful proxy for the lowest cost. The received design approval covers a total power of 11-15MW on one floater, and drawing on the learnings from certification and the relative ease of further scaling-up by using two turbines as mentioned above, the fully commercial design for Enerocean’s future pipeline has since been upgraded to 20+ MW.
One benefit of the certification process of being on known ground, apart from reaping the benefits of decades of design and operational excellence, is the availability of advanced software simulation tools, which, with very high confidence levels, can predict how a structure will behave in any kind of weather.
This is why classification societies consider a floating wind design based on well-known design principles and having reached a detail and maturity level sufficient for achieving a Basic Design Approval as having a high TRL. Experience in wave-tank testing and the optimisation of scale models allows the certification body to calibrate and verify floater motions and loads for further confidence and the technology developer to move forward with greater confidence in realising.
For Enerocean, this certification represents the completion of a comprehensive assessment of critical elements of the W2Power design, which is currently moving to full-size commercial installations.
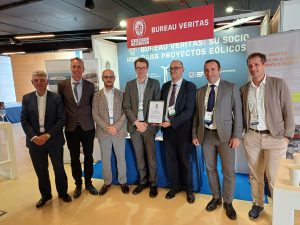
The design assessment that BV has now completed on Enerocean’s W2Power ensures that best practices have been adequately implemented. The review includes documents (drawings, analysis and specifications) demonstrating that the design complies with regulations and design codes – with a view to its later construction. Documentation includes the design methodologies and preliminary analyses, illustrating the analytical method used in the detailed design phase.
In a situation where a nascent industry is struggling to make its mark versus investors and policymakers, the growing importance of high-level certification to new promising technologies on the path to commercialisation is a big step forward.
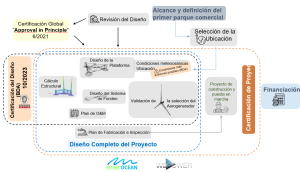
Fig. 6. Process used by BV and Enerocean leading to design certification, 2021-23
For the offshore wind industry, it is key in the following years to ensure that public incentives and commercial investments go to technologies that have proven their technical performance and simultaneously possess a natural ability to address the cost-reduction challenges successfully.
References
- Ørsted (Jørgen Skovmose Madsen, Head of EU Regulatory Affairs)
- Wind Europe, 12 January 2024
- Global Energy Monitor: A Race to the Top, China 2023
- US DoE report on floating wind 2023
- ‘Global floating offshore wind pipeline grows by one-third over 12 months’, Renewable UK
- ‘Floating wind’s embryonic state means small contribution this decade’, Windpower Monthly
- Deployment of innovative renewable energy technologies to 2030, Report by EUREC and 1-Tech
- France earmarks EUR 4.12 billion for 2024 floating wind tender, offshorewind.biz
- Market analysis by Norton Rose Fulbright December 2023
- BV gives design approval for Enerocean’s W2Power floating wind solution, Offshore Engineer