Jamie Boyd, Co-Spokesperson for the FASER and FASERν experiments at CERN, discusses the aim of the projects and the potential impacts of recent discoveries on the field.
In March 2023, the Forward Search Experiment (FASER) published its first results, detecting collider neutrinos for the first time in an experiment environment.
FASER is one of the most recent particle physics experiments established at the Large Hadron Collider at CERN. The experiment started taking data in July 2022, and stopped taking data, for this year, in July 2023.
Designed to discover and study the elusive, weakly interacting particles that can be produced in collisions, the FASER experiment could help to solve some of the biggest mysteries in particle physics. By putting predictions to the test, the experiment refines our understanding of concepts such as dark matter, and enables us to confirm, exclude or possibly introduce predictions. Its subdetector, FASERν, studies interactions between high-energy neutrinos, developing our understanding of fundamental physics.
The Innovation Platform spoke with CERN Research Scientist and FASER Co-Spokesperson, Jamie Boyd, to learn more about the FASER and FASERν experiments, as well as the potential implications of recent discoveries on the field.
Can you explain the FASER and FASERν experiments and their objectives?
The FASER experiment was designed to search for hypothetical new particles that could be produced in the Large Hadron Collider (LHC) collisions. FASER is positioned in a special location, different to other experiments at the LHC, to be sensitive to new particles that could potentially be produced, but not seen, by other experiments.
The search is motivated by models that attempt to explain dark matter. Thanks to observations in space, we know about the existence of dark matter, and it can be explained by models that would potentially show new particles at the Large Hadron Collider.
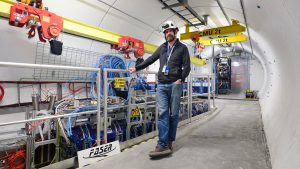
FASER is also designed to study high-energy neutrinos that are produced in LHC collisions. FASERν is a sub detector and is part of the FASER experiment designed to detect and study neutrinos.
Neutrinos are part of the standard model of particle physics, the theoretical framework that has been well-validated by experiments. However, they’ve never been studied when produced at a particle collider. At a collider, we can study the highest-energy neutrinos ever produced in a laboratory experiment. Though higher energy neutrinos have been studied coming from astrophysical sources, it has traditionally been difficult to know where they come from or what is producing them.
In the LHC collisions, we know the energy of the colliding protons, and consequently, we know how the neutrinos are produced, the energy they have, and can therefore study their interactions.
Typically, a new facility would be required to study such neutrinos, bringing associated large costs while also being time and labour-intensive. FASER allows us to take advantage of the neutrinos already being produced at the LHC. Utilising an existing facility in this way is very efficient, and we are truly maximising the physics that can be extracted from it.
What progress has been made since you began taking data in 2022?
We started taking data at the start of Run 3 of the LHC, in July 2022, and data was then taken continuously while the LHC was colliding protons, until November 2022. The process then began again in March 2023.
Using the data from 2022, FASER released two results for the Winter Conferences in March 2023. One of these was the search for a possible new particle that could help explain dark matter, the dark photon. An analysis was conducted to search for this dark photon, but unfortunately, it was not found. However, this did enable us to exclude certain dark photon lifetimes and masses, and consequently, refine the allowed parameters in the model.
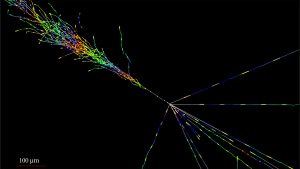
Perhaps more exciting was the detection of neutrinos. Although we are familiar with these particles, we have yet to see collider neutrinos in an experiment environment, with these results marking their detection for the first time. Both the detector, and the experiment as a whole, operated very well. That neutrinos were highly visible in our data gives us conduct very interesting neutrino studies as more data is collected.
Why is it important that we understand neutrinos?
Neutrinos are a very elusive, fundamental particle in the standard model, interacting so weakly that they are hard to capture and study. Although, there’s a huge number of them, experimentally, they are difficult to measure. As a result, they have not been well understood.
The traditional method of studying neutrinos requires shooting a beam of particles into a fixed target that then intersects the beam and produces a shower of secondary particles. Among these secondary particles are neutrinos. However, such neutrinos are typically produced at between 100 and 1000 times lower energy than the ones observed in FASER.
There are several models beyond the standard model, designed to answer different types of questions in particle physics, and these can modify how neutrinos interact at high energy. With FASER, we will be able to constrain these models with data for the first time, using an unexplored energy.
Neutrinos from astrophysical sources are also important for studying and increasing our understanding of the universe.
Ice Cube is an experiment based in the Antarctic that uses the ice as a target to measure neutrinos coming from space. This enables us to look for dark matter, black holes, and effects yet to be discovered. These are very high-energy neutrinos, and understanding their interactions is an important element of utilising Ice Cube data to learn about fundamental physics.
The measurements of neutrinos made at FASER will help with this endeavour, and bring us closer understanding some of the most mysterious aspects of the universe.
In July, the teams at CERN quickly and expertly discovered and solved a leak in the LHC, did this affect the FASER experiment?
Although the leak was quickly fixed, the data produced since its repair has targeted experiments other than FASER. Therefore, FASER essentially finished taking data – for 2023 – earlier than expected, as a result of the leak.
However, we are operating at the very cutting edge of technology. The magnets of the LHC are the coldest place in the Universe, and operate under extreme vacuum. Problems such as these are therefore normal and anticipated.
What are the challenges faced when conducting a project like this?
The FASER location presents the biggest challenge, as it is very specific and difficult to access. The access route is narrow and, in some areas, it is highly radiated. It also requires walking along the LHC, which can’t be done while the accelerator operates.
These factors necessitate a robust detector rarely in need of maintenance. So far, this has been successful. The detector has worked as expected, and no intervention has been required during beam operation.
An experiment designed to operate in space is constructed under the assumption that it is inaccessible, meaning reliability is a necessity, and there must be contingencies in place that enable the project to operate, should parts break. The same principle is generally applied at CERN.
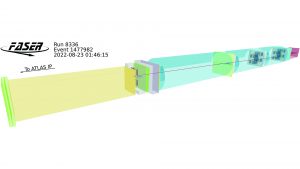
To our advantage, at CERN there is an option to repair and access the experiments if necessary, which provides a degree of leeway. However, to do so interrupts the operation of the LHC and has ramifications across the entire CERN physics programme. Thus, to minimise intervention, the experiments are designed and built to be highly reliable and robust.
What does your recent neutrino discovery, with FASER, mean for the experiment?
FASER is a new experiment, constructed over the recent long shutdown and only in operation for little over a year. That the experiment operated as expected, and collider neutrinos were observed so quickly is really promising. The operations ran smoothly and the detector performance was excellent, a factor not guaranteed in an experiment of this kind.
As a young, small team, the effort required to achieve a project of this scale was significant, and therefore, the results are particularly rewarding. The results demonstrate the experiment’s successful design, which is encouraging news for the future of our operations, and enabling us to continue as anticipated.
Why is it such a significant development in the field?
Regarding the field in general, this particular result is a proof of concept. It proves we are capable of studying neutrinos at the LHC in the high-energy regime. As FASER takes more data in the next years, we will have the capacity and time to analyse our results more thoroughly, to extract useful – and possibly entirely novel – information about high-energy neutrinos.
The project marks an important new chapter in this area of physics, the results of which will be applicable to astrophysics and particle physics. These results are the first step towards utilising this experiment to better understand fundamental physics.
Please note, this article will also appear in the sixteenth edition of our quarterly publication.