Advancing the state of the art in nature-based solutions, especially using wood, to accelerate the adoption of regenerative sustainability.
InnoRenew CoE’s mission is to advance the state of the art in nature-based solutions, especially using wood, to support and accelerate the adoption of regenerative sustainability through material and processing advances, developing design and engineering solutions that promote sustainable, healthy built environments, and engineering living materials to bring the function and capabilities of living organisations to buildings. InnoRenew CoE brings this work together in restorative environmental and ergonmic design (REED), its approach to using nature-based solutions in the built environment for health and sustainability.
InnoRenew CoE’s research capacities are unequivocally unique and well-suited to the current and anticipated trends in research.
The organisation has a unique set of state-of-the-art equipment that is ideally suited to research on nature-based solutions for use in the construction sector, consumer products, and related areas. The scientific staff is similarly unique. The interdisciplinary team comprises material scientists, computer scientists, engineers, architects, psychologists, health researchers, acousticians, and biologists. It was designed following the design guidelines we have developed for wood-based construction (REED), has state-of-the-art monitoring systems, utilises innovative wood products, and its carbon impact was offset by planting 3000 trees in the local area.
State-of-the-art laboratories
The facility houses nine state-of-the-art laboratories and research equipment.
- The Human Health Laboratory — designed to assess human well-being and performance under different indoor environmental conditions;
- Composites Laboratory — houses equipment for the preparation of bio-based composite materials;
- The Physical Testing Laboratory — assesses the static, dynamic, and creep behaviour of elastic, plastic, and viscoelastic materials as well as perform in-situ testing of the built environment;
- The Characterisation Laboratory — offers morphological, physicochemical, and rheological characterisation of bio-based materials;
- The Microscopy Laboratory — has microscopes and sample preparation devices to analyse a range of materials at micro- and nanoscale;
- The Acoustic Laboratory — fully equipped to perform research in architectural and building acoustics, noise control, structural dynamics, and vibrations;
- The Advanced Manufacturing Laboratory — a technological hub providing scientific support for a broad range of bio-based businesses as they adapt to Industry 4.0 and beyond;
- The Engineered Living Materials Laboratory— implements biomimetic principles for the development of new materials and modification processes; and
- The Workshop and Machine Shop — service provider for other laboratories, enabling in-house preparation, conditioning, and storage of experimental samples and prototypes.
The InnoRenew CoE strives to become the driver of change in regenerative sustainability through scientific advances that will bring a paradigm shift, leading to the decarbonisation of the building stock and a built environment that is a healthier, more sustainable, and beautiful ecosystem. These goals will be achieved through continued scientific excellence, innovation of the ecosystem, co-creation activities, industry collaboration, and policy development. These achievements will be transferred to society through activities such as policy outreach, training and life-long learning, open science, and others that follow the key impact pathways across scientific, economic, and societal domains.
InnoRenew CoE’s main areas of research are materials, advanced manufacturing, buildings, information processing, health, and creativity and society. In each of the areas, we have clearly defined goals and advances we aim to make.
Materials
Goal: Designing, developing, and testing a new generation of functional materials with enhanced properties and extended service life that supports the uptake of renewable materials in the built environment.
Advances in the field we expect to make:
- Engineered living materials – materials composed, either entirely or partially, of living cells able to replicate the function and capabilities of living organisms;
- Wood modification – wood with enhanced properties, embedded new functionalities, and extended service life; and
- Composite materials – value-added composite materials from underutilised wood species and other biobased resources for use in high-performance applications
Advanced manufacturing
Goal: To progress regenerative sustainability transformation technologies across the entire value chain by implementing concepts from Industry 4.0 and 5.0 along with the latest technological advances to improve resilience and achieve the construction sector’s sustainability goals.
Advances in the field we expect to make:
- Identify flexible robotic applications for SMEs in the timber construction sector;
- Promote better use of production data for process optimisation and increase economic and environmental sustainability;
- Encourage industrial collaboration with academic partners toward the development and implementation of RDI activities; and
- Evidence advantages of digital twin concepts and implementation of virtual tools (such as BIM) along the whole value/transformation chain
Buildings
Goal: Building efficiently to create safe, healthy, productive, and restorative built environments that embrace renewable materials, innovative design, and advanced building technologies.
Advances in the field we expect to make:
- Sustainability of buildings;
- Room and building acoustics; and
- Timber and hybrid building structures
Information processing
Goal: Integrating digitalisation, optimisation, and knowledge discovery in complex information processing frameworks to support all life-cycle phases of renewable materials and buildings.
Advances in the field we expect to make:
- Building monitoring — secure, privacy-aware decentralised monitoring by distributed systems and networks. Highlighted applications are air quality monitoring and structural health monitoring;
- Optimisation for regenerative sustainability — efficient computational optimisation models and methods for practical challenges with sustainability aspects such as environmental impact or energy efficiency;
- Scientific computing and AI – integration of network science methods, simulations, and AI for social science problems in built environment and renewable materials research;
- Survey methods — improvements in survey methods to improve data accuracy on user preferences and needs regarding the built environment; and
- Digital twins for sustainable environment — multi-purpose digital twins for smart built environment (buildings and districts) with advanced analytics layers
Health
Goal: To understand the human relationship with the built environment from the psychosocial and physiological perspectives, and to determine how to improve human health through the built environment and related interventions.
Advances in the field we expect to make:
- Acquiring new knowledge linking building design and material selection to occupant health and wellbeing;
- Improved health outcomes for building occupants;
- Evidence for design solutions to improve health and wellbeing in the built environment; and
- Links between activity behaviours and health
Creativity and society
Goal: To promote regenerative building solutions and business practices throughout the renewable materials sector, and engage society in the development of regenerative sustainability solutions and practices through embracing creativity and knowledge transfer.
Advances in the field we expect to make:
- Intensifying the institute’s knowledge transfer to diverse audiences;
- Development and utilisation of new knowledge management and transfer methods;
- Active engagement with the public, policy makers, and educators;
- Development of novel user-led design methods for products and the built environment;
- Innovation in learning and training approaches for learners of all ages;
- Applications and advances in life-cycle analysis;
- Protection, renovation, and utilisation of cultural heritage objects with renewable materials; and
- Methods and solutions for environmental, sustainability, and governance (ESG)
Scientific advancements in regenerative sustainability
InnoRenew CoE has made several significant advancements in the past years. One of the most notable advancements was the development of engineering living materials (ELMs) capable of interacting and responding to environmental conditions, considered the most relevant contemporary revolution in materials science and engineering (Sandak, 2023). ELMs are defined as materials composed entirely or partly of living cells. Due to their unique functionalities and outstanding properties, they outperform current examples of ‘smart materials’ (Fig. 1).
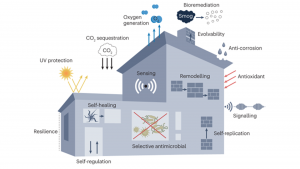
Significant progress was made in advancing new processes and product quality control procedures (Sandak et al, 2019). This activity allowed an understanding of the physical and chemical characteristics of modified biomaterials. Different multivariate process control tools were adopted for state-of-the-art wood modification processes, allowing real-time and in-line monitoring of processes.
Another ongoing research line is biochar as an additive to composite materials. Biochar (activated carbon) is currently used for the purification of water, soil amendments, etc., but it can also be used for cleaning indoor air and enhancing bonding in composites. Research projects are investigating the manufacture of biochar (Zouari et al, 2023) from agriculture residues for use in adhesive systems, VOC absorbing coatings for indoor environments, and as an additive to concrete to improve adhesion with natural fibre reinforcements (Fig. 2). Biochar was also used as an additive to improve adhesion between wood particles in polylactic acid (PLA) blends to be used in food packaging (Zouari et al, 2023, Zouari et al. 2022, Esakkumuthu et al, 2022). As this is a bio-based and biodegradable material, it is of great value in a new era of bio-based and biodegradable products.
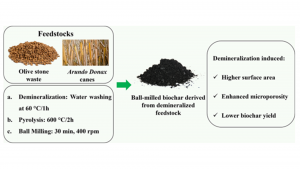
Wood supply chain optimisation has been active over the past years, but there were only minimal results in optimising processes in the waste wood supply chain. We focused on locating the accumulation centres in the supply network in such a way as to minimise the costs of transporting waste wood from accumulation centres to processing facilities (Egri et al, 2020). Besides transportation, we also studied economies of scale (Egri et al, 2023) as well as the robustness of the network, in case of ‘the breakdown of facilities’ (non-operating accumulation centres). In co-operation with our research partners, we have produced efficient mathematical optimisation models with nature-based solutions.
InnoRenew was also responsible for the development of a macro-level optimisation model in Slovenia that allows for the multi-objective optimisation of wood volumes for processing across the entire forestry, wood and paper sector for long-term policy co-ordinated with the broadest range of stakeholders (Schau et al, 2023). This innovative approach can elevate industrial optimisation models for macro-level policymaking. The model is based on development needs, the businesses and investment plans of different stakeholders, and a holistic assessment of different scenarios for developing logging, processing, and utilisation of wood in Slovenia.
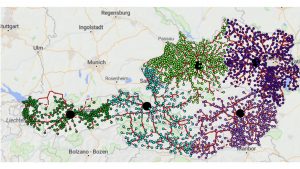
A new alliance for new regenerative sustainability research
InnoRenew CoE, together with the University of Primorska, Oregon State University, and the University of Helsinki, have established a research alliance for sharing information on mass timber building performance. In the frame of this co-operation, we are monitoring data from three mass-timber projects in three different climate zones. One of the alliance’s goals is to develop a unified framework for data collection, sharing, and analytics by implementing pilots using these mass timber projects (Riggio et al., 2023). The main difficulties of data integration are due to differences in data collection methods, equipment, and techniques, as well as variations in the number and types of monitored locations and measurement periods. A data integration framework based on ‘avatars’ (decentralised computing agents) has been suggested by InnoRenew researchers (Riggio et al., 2022), and it was compared to state-of-the-art structural health monitoring (SHM) solutions.
As we spend most of our time indoors, indoor air quality (IAQ) monitoring is essential to ensure healthy living. It also contributes greatly to improving building performance and reducing energy consumption. Current IAQ monitoring systems are generally based on WSNs and organised centrally with cloud data management infrastructure. These solutions have serious limitations in data privacy and security. Decentralised approaches such as edge computing offer a good alternative, but distributed computing still faces privacy issues — to name just one: the data collected requires basic security protection as it describes physical phenomena that can reveal activities in the monitored space. For example, changes in CO2 and temperature can indicate human presence in a room. The privacy-aware and secure decentralised IAQ monitoring system developed by InnoRenew researchers with project partners (Mrissa et al., 2022) provides an advance beyond the state-of-the-art that uses the data while providing security and privacy for those in the monitored building.
Through other studies, we have investigated the complex interplay between indoor environments and human well-being, focusing on the important role that material selection and ergonomic settings play in fostering human well-being. One of the crucial outcomes that emerged from our research was that using wood in offices shows promise for reducing stress and improving stress recovery in occupants. One of the studies, which examined stress responses in office settings, has yielded important insights about designing spaces for wellbeing. By comparing offices treated with oak and walnut wood with control settings, we have shown that the presence of wood can lower people’s stress levels, thereby presenting architects and designers with an option to design environments that can support the well-being of building occupants. This was one of the first robust studies that showed wood can be a promising addition to indoor spaces to improve people’s well-being (Burnard and Kutnar, 2020).
References:
Sandak, A, (2023). Engineered living materials for sustainable and resilient architecture. Nature Reviews Materials, 8, 357–359.
Sandak, J, Orlowski, K A, Sandak, A, Chuchala, D, & Taube, P, (2019a). In-Line Measurement of Wood Surface Roughness. Drvna Indistrija 71(2): 193-200.
Zouari, M, Mikuljan, M, & Schwarzkopf, M, (2023). Impact of biochar on the fungal decay resistance of hemp-polylactic acid composites against wood basidiomycetes. Frontiers in Materials, 10, 1129303.
Zouari, M, Devallance, D B, & Marrot, L, (2022). Effect of biochar addition on mechanical properties, thermal stability, and water resistance of hemp-polylactic acid (PLA) composites. Materials, 15(6), 2271.
Esakkimuthu, E S, DeVallance, D, Pylypchuk, I, Moreno, A, & Sipponen, M H, (2022). Multifunctional lignin-poly (lactic acid) biocomposites for packaging applications. Frontiers in bioengineering and biotechnology, 10, 1025076.
Egri, P, Dávid, B, Kis, T, & Krész, M, (2023). Robust facility location in reverse logistics. Annals of Operations Research, 324(1-2), 163-188.
Egri, P, Dávid, B, Kis, T, Krész, M, (2020). Robust Reverse Logistics Network Design. In: Golinska-Dawson, P, (eds) Logistics Operations and Management for Recycling and Reuse. EcoProduction. Springer, Berlin, Heidelberg.
Schau, E M, Gavrić, I, Šušteršič, I, Prelovšek Niemelä, E, Dávid, B, Pečnik, J G, … & Tavzes, Č. (2023). Modelling the Slovenian Wood Industry’s Response to the Greenhouse Gas Paris Agreement and the EU “Fit for 55” Green Transition Plan. Sustainability, 15(10), 8376.
Riggio, M, Mrissa, M, Krész, M, Včelák, J, Sandak, J, & Sandak, A, (2022). Leveraging structural health monitoring data through avatars to extend the service life of mass timber buildings. Frontiers in Built Environment, 8, 887593.
Riggio, M, Vcelak, J, Kaitaniemi, P, Barbosa, A R, Hrovatin, N, Sandak, A, … & Alakukku10, L (2023). Federated use of hygrothermal monitoring data in mass timber buildings: opportunities and challenges. In World Conference on Timber Engineering (WCTE 2023) (pp. 3896-3904).
Mrissa, M, Tošić, A, Hrovatin, N, Aslam, S, Dávid, B, Hajdu, L, … & Kavšek, B, (2022). Privacy-aware and secure decentralised air quality monitoring. Applied sciences, 12(4), 2147.
Burnard, M D, & Kutnar, A (2020). Human stress responses in office-like environments with wood furniture. Building Research & Information, 48(3), 316-330.
Please note, this article will also appear in the sixteenth edition of our quarterly publication.