The IceCube Observatory could unlock the mysteries of the Universe through its advanced neutrino discoveries.
Neutrinos are tiny, nearly massless particles that rarely interact with their surroundings, passing through us unnoticed. These ‘ghost-like’ particles hold the key to unlocking the mysteries of the Universe. Very large detectors, such as the IceCube Neutrino Observatory located at the South Pole, need to be built to discover elusive particles.
Building the strangest detector in the world
In the early 1960s, only a few years after the experimental observation of neutrinos by Cowen and Reines, the concept of very large Cherenkov neutrino detectors to measure cosmic neutrinos was conceived. While pioneering efforts were made to realise a large Cherenkov detector in water, they were met with challenges.
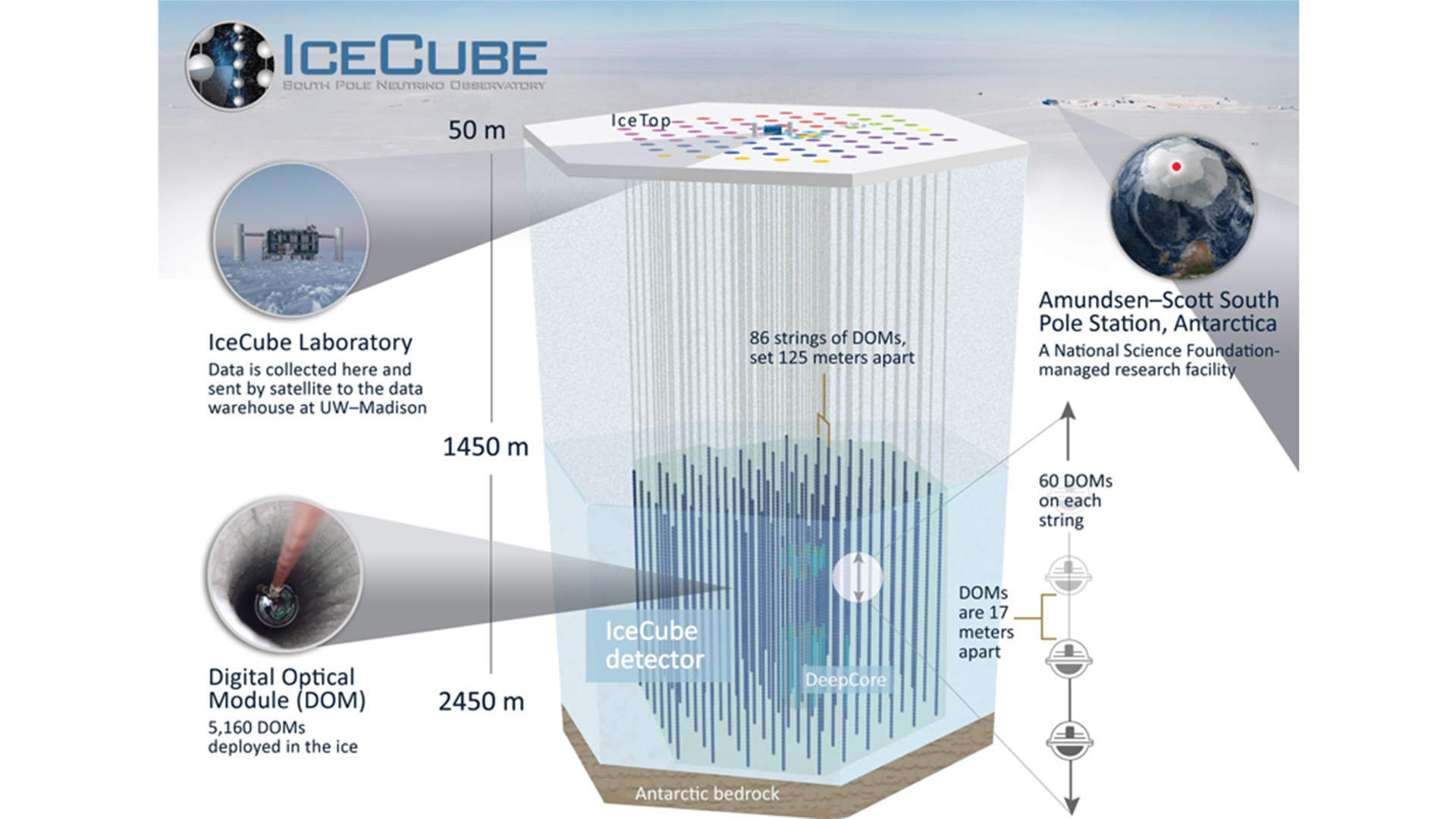
In the early 1990s, the idea to build a large neutrino Cherenkov telescope in ice became real, and the observation of atmospheric neutrinos of TeV energies at the South Pole set the stage for IceCube1. The construction of IceCube was a formidable undertaking. Over the course of seven Antarctic summer seasons, which consisted of around ten weeks each, 86 holes of 55cm diameter were drilled to a depth of 2500m.
Those water-filled holes were instrumented with over 5000 optical sensors – ten-inch photomultiplier tubes (PMTs) inside glass pressure-resistant spheres – now frozen in place. The detector encompasses one Gigaton of optically transparent glacial ice, simultaneously serving as a massive target for neutrinos of atmospheric and cosmic origin and as a Cherenkov radiator medium. The completed IceCube Neutrino Observatory has been in operation since 2011. 98.7% of the sensors are still in continuous operation 12 years after IceCube construction was completed. Today, the international IceCube collaboration consists of 350 scientists at 58 institutions.
In 2013, after only two years of operation, IceCube made its first discovery with the detection of a cosmic neutrino flux. These cosmic neutrinos can be differentiated from other types, such as those produced in the Earth’s atmosphere, largely thanks to their high energies. The cosmic neutrino spectrum dominates the atmospheric neutrino background at approximately 100 TeV and extends up to, and possibly beyond, the PeV scale.
The IceCube Collaboration recently reported observing an astrophysical point source of TeV neutrinos. IceCube counted an excess of 81 neutrinos at TeV energies from the direction of the nearby active galaxy NGC 1068,2 which hosts a massive black hole in its centre. With IceCube, neutrinos have become a firm part of a new discipline called multi-messenger astrophysics. To understand astrophysical sources, multiple messengers are required, including photons over a wide range of energies, cosmic rays, neutrinos, and gravitational waves.
The future of IceCube – Phase 1
The energy threshold of the water/ice Cherenkov detectors depends on the coverage of PMTs per kiloton of water and ice. For extremely large detectors, the optical quality of the medium becomes increasingly essential. Underground water Cherenkov detectors, such as the pioneering 6KT Irvine-Michigan-Brookhaven in the US and the 50KT Super-Kamiokande detector in Japan, typically have on the order 1,000 PMTs per kiloton for an energy threshold of a few MeV.
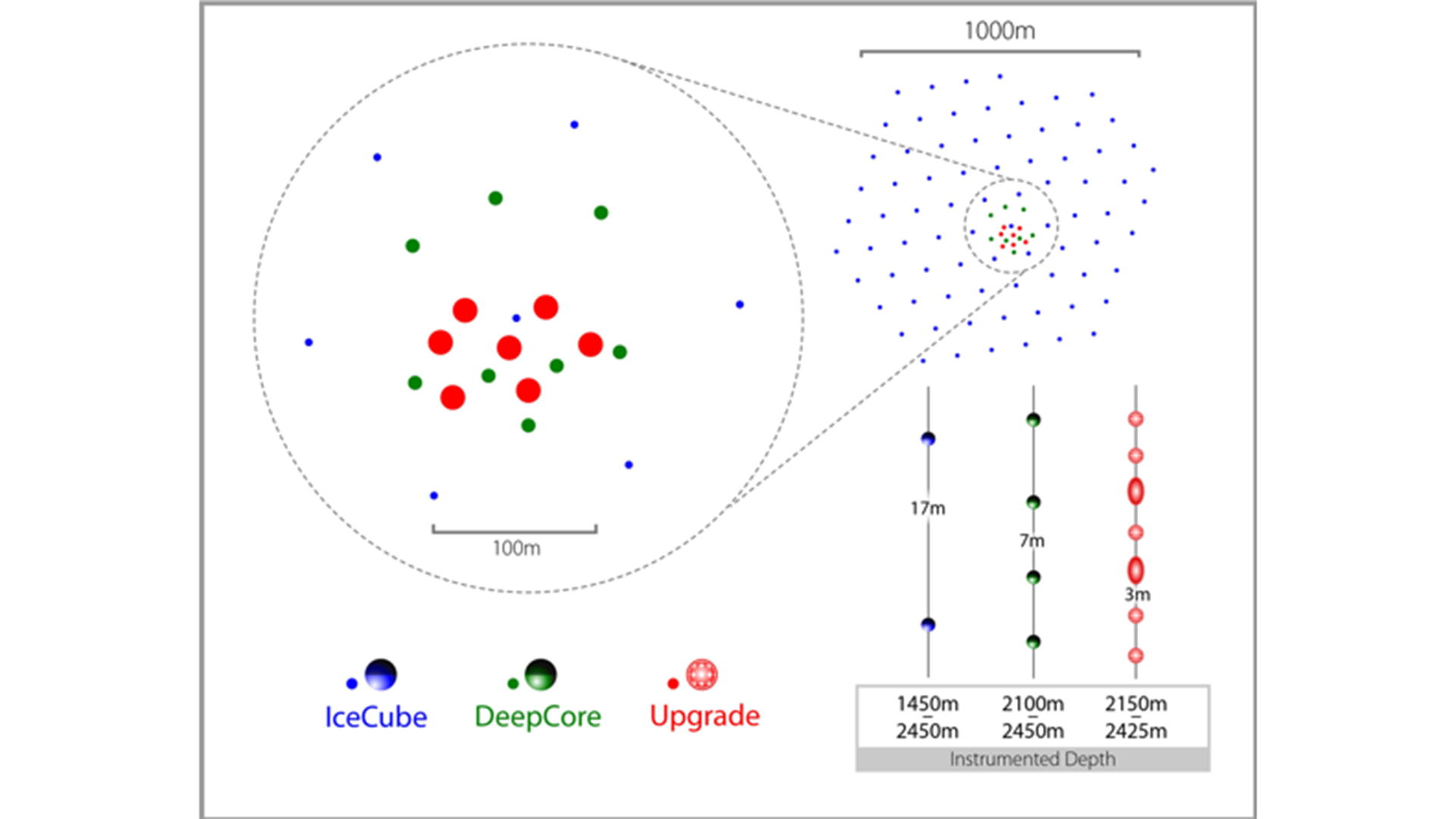
By comparison, most of the IceCube detector has around five photomultipliers per one million tonnes of ice – enough to detect charged particles at a threshold of a few 100 GeV. An exception lies at the centre of IceCube, referred to as DeepCore. The DeepCore region comprises 10 Mtons of ice that is more densely instrumented with 50 PMTs/Mton. Correspondingly, DeepCore has higher sensitivity to dimmer, lower-energy events as low as 5 GeV. In addition, the surrounding IceCube strings provide a highly effective active veto against the cosmic ray muon background.
This has opened up a range of exciting, high-impact physics studies in this energy regime. Neutrino oscillations have been well measured by particle astrophysics experiments such as Super-Kamiokande1. DeepCore follows in this tradition4, bringing several enticing new features. Its sheer size provides a neutrino data set of enormous statistical power; with a fiducial volume of ten Mton at five GeV, DeepCore collects nearly 100,000 atmospheric neutrino events annually.
Now, construction is underway for an additional seven holes filled with optical sensors in the centre of IceCube’s DeepCore region that pushes the energy threshold of the facility an order of magnitude below that of DeepCore. The IceCube Upgrade takes a big step in focusing on oscillation measurements unique to the atmospheric neutrino beam. This is possible to a large extent thanks to new multi-PMT digital optical modules, providing better directional information and more than double the photocathode area per module compared to traditional IceCube DOMs.
The sensors will be fully integrated into the IceCube data acquisition system. Every string will contain as many as 90 sensors at depths from 2150m-2450m. For comparison, an IceCube string contains 60 sensors over one kilometre. The neutrino physics region of the Upgrade will have 630 sensors in a volume of 2.7Mtons, with a PMT cathode coverage/Mton of 140 times larger than IceCube. The two primary optical sensors for the Upgrade are the mDOM and the D-Egg (Fig. 3). A total of 402 mDOMs and 277 D-Eggs will be installed on the seven strings.
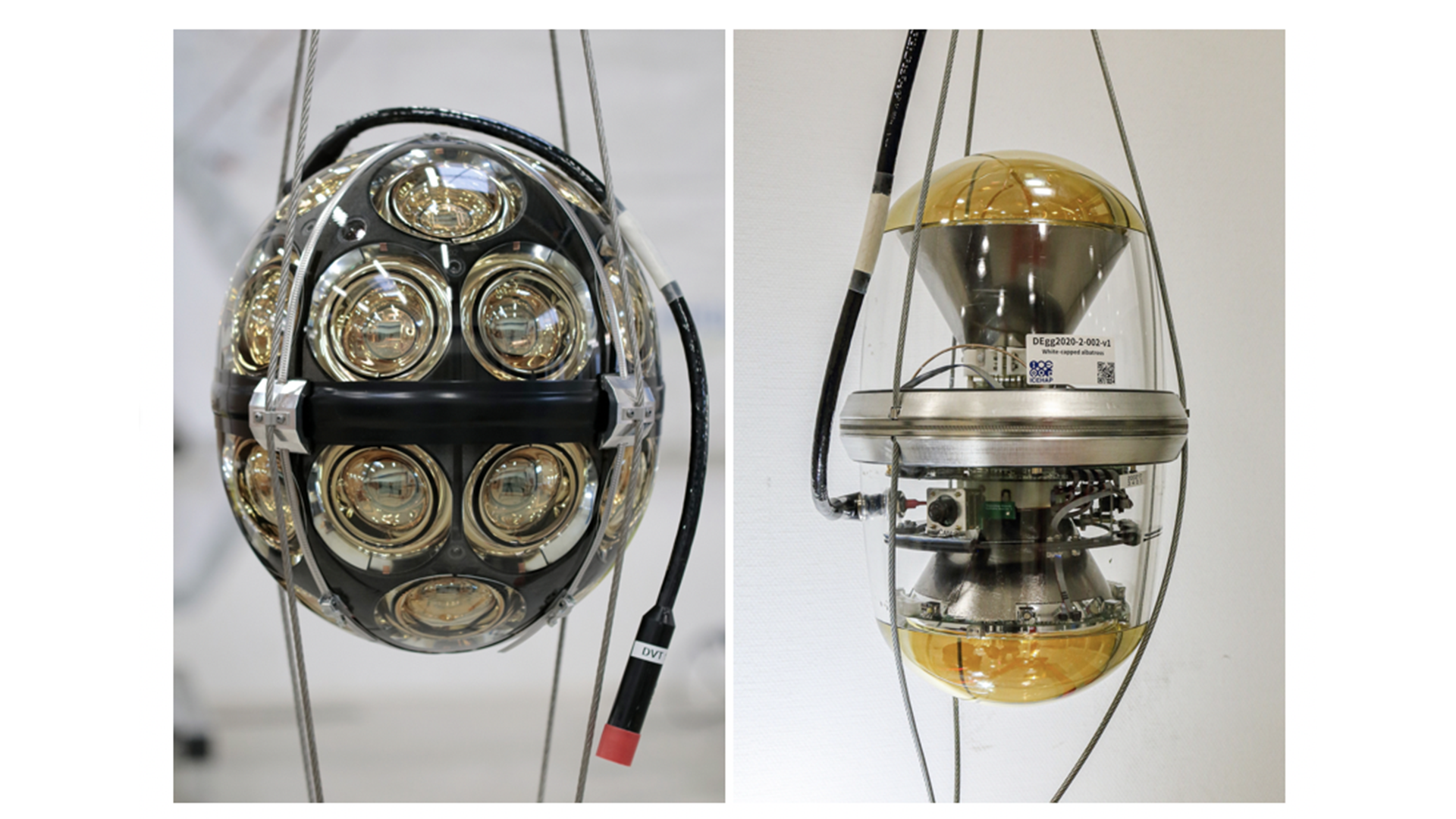
The new strings will dramatically improve IceCube’s neutrino oscillation physics reach by lowering the energy threshold to below five GeV and improving reconstruction resolutions. The strings will also contain additional calibration instruments. A small portion of the sensors will be deployed at shallower and deeper depths for calibration, atmospheric muon vetoing, and high-energy reconstruction.
Neutrino oscillation physics with atmospheric neutrinos
Neutrinos come in three different types or flavours: electron, muon, and tau-neutrinos. One of the most fascinating and unexpected discoveries in recent decades has been that neutrinos have mass – something not predicted in the Standard Model of particle physics.
Moreover, the different neutrino flavours are quantum super-positions of unequal mass states. As the mass states propagate at different speeds, minor phase differences accumulate between their mass and flavour states, ultimately leading to an effect where the observed neutrino flavour appears to change back and forth over time, referred to as oscillations. The oscillation probabilities depend on the ratio of the distance travelled and their energy, L/E.
Atmospheric neutrinos are abundantly produced in the Earth’s atmosphere as decay products of particles created in cosmic ray interactions and come in mostly muon and electron flavours. As they pass through the Earth, the relative abundances of neutrino flavours are modulated through oscillations. The primary effect that can be observed for GeV-scale neutrinos over length scales on par with Earth’s diameter is the oscillation of muon neutrinos into tau neutrinos. The strength and frequency of this modulation are characterised by the so-called atmospheric oscillation parameters, which must be experimentally determined as there is no predominant theoretical prediction for their values.
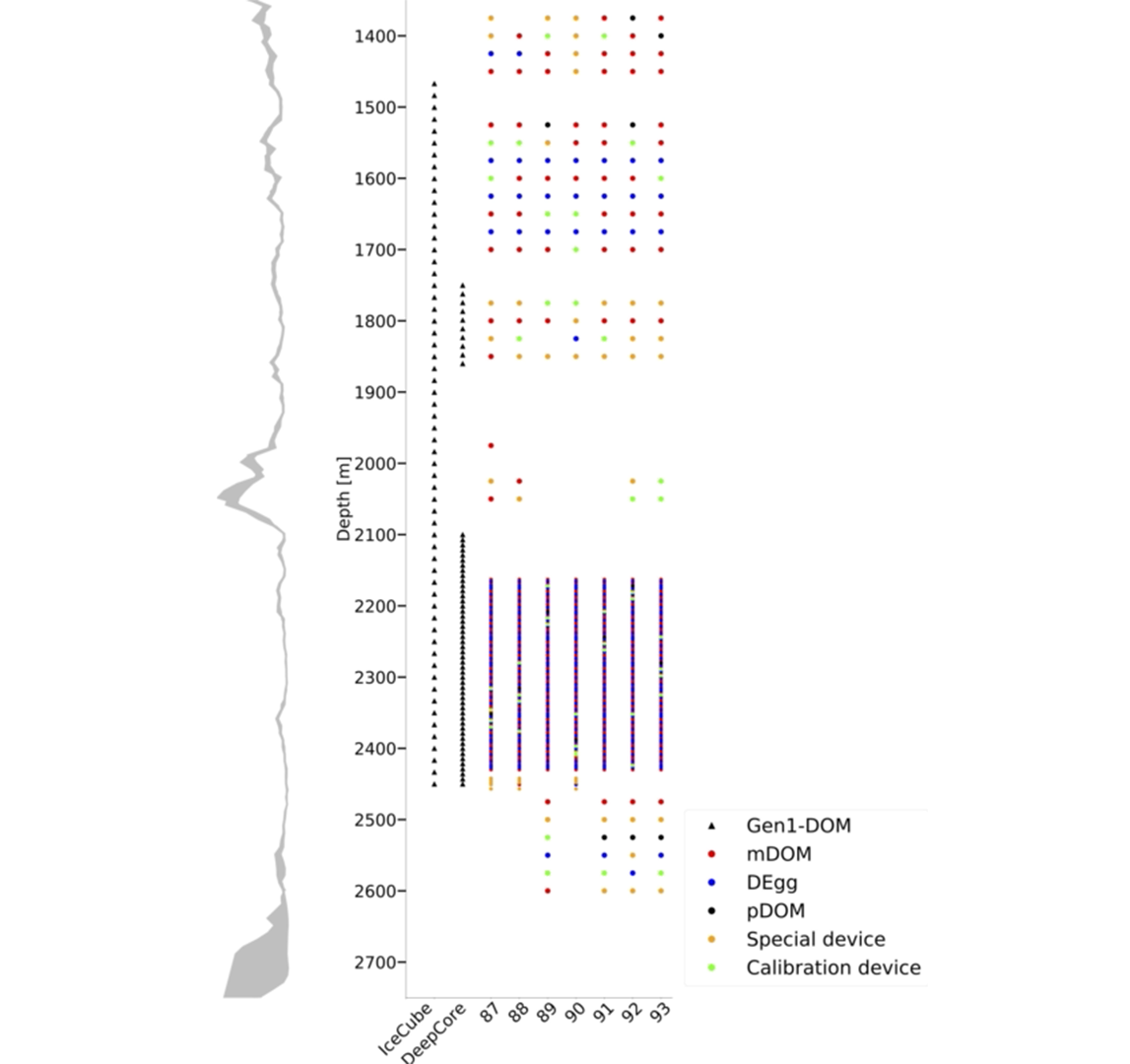
Previously published measurements of atmospheric neutrino oscillations4,6 demonstrate the physics potential of IceCube DeepCore’s massive fiducial volume and the background reduction offered by the surrounding IceCube sensors, which form a strong veto for the cosmic ray muon background. The ban from the surrounding IceCube strings suppresses the background from cosmic ray muons by a factor of 10−7, such that the remaining background is insignificant compared to the neutrino flux – equivalent to a detector with an overburden of more than 6km of rock.
Fig. 5 shows the anticipated measurements of the atmospheric oscillation parameters for three years of IceCube Upgrade exposure, compared to existing IceCube DeepCore measurements and other atmospheric and accelerator neutrino measurements.
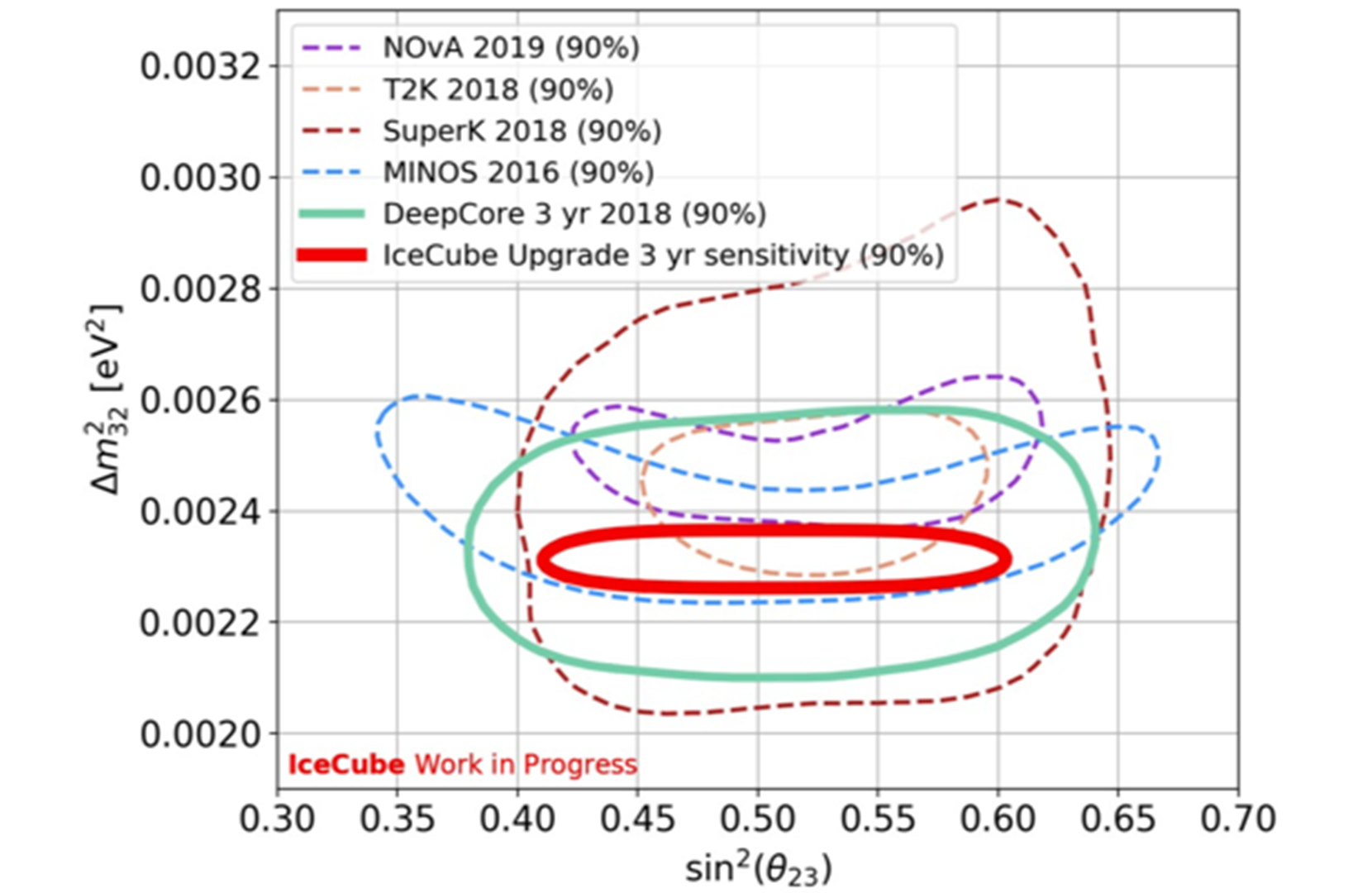
The IceCube Upgrade promises a substantial improvement over existing measurements. Moreover, the Upgrade is a significantly more sensitive instrument to probe beyond Standard Model physics via neutrino oscillations. It can be used to observe the disappearance of muon neutrinos and test for a commensurate appearance of a tau neutrino flux. By probing both sides of this transition, the upgrade can directly test whether some new physics or particles at play affects the rate of observed tau neutrinos in a model-independent way.
After one year of operation, the Upgrade will measure the tau-neutrino flux normalisation to a precision of 10%, a factor of three better than any other current experimental constraint, including DeepCore’s. The expected results do not yet leverage cutting-edge techniques being concurrently developed with IceCube and DeepCore, principally using Artificial Intelligence and Machine Learning techniques, that are certain to elevate the ultimate performance of the Upgrade beyond what is estimated.
Improving IceCube through precision calibration
Increasingly, systematic uncertainties are becoming a limiting factor for determining angular resolution, for measurements of atmospheric neutrino oscillations and pointing cosmic neutrinos back to their sources. The accuracy of our event reconstruction is limited by our knowledge of the optical properties of the glacial ice, refrozen hole ice and the in situ response function of the sensors themselves.
The IceCube Upgrade presents an opportunity to apply the knowledge gained after 15 years of operating IceCube and make progressive refinements to numerical models of the ice and the detector. Using new calibration methods will improve our understanding of the glacial ice stratigraphy, which records Earth’s climate history going back nearly 100,000 years (see left panel of Fig. 4).
This will include insights into how the glacial flow and underlying bedrock structure influence the three-dimensional topology of ice layers and their birefringence effects. The high density of optical instrumentation in the Upgrade will be ideal for probing scattering in the deep ice for the first time over distance scales shorter than the geometric scattering length. As with IceCube, each new optical module features a series of pulsed LEDs that can calibrate the ice properties that impact light propagation. Modules will also contain small, fixed-focus cameras, offering a complementary method for ice calibration through transmission and reflective photography. Rotating, variable-focus video cameras will also be deployed in a few choice locations for ice measurements alongside similarly steerable, collimated ‘pencil-beam’ lasers that probe scattering behaviour on short baselines.
The primary goals of the calibration subsystems are a determination of relative module optical efficiency to within 3%, a halving of the ice model fitting errors associated with hole ice, a detailed understanding of ice anisotropies, and measurement of ice properties below the instrumented volume of IceCube. With the Upgrade, the calibration of IceCube will be significantly improved, allowing for a re-analysis of IceCube’s unique ∼ ten-kilometre^3yr exposure data set.
Research and development
A third high-level goal of the IceCube Upgrade is to serve as a research and development platform for a much larger future upgrade, the IceCube-Gen2 detector7. Advances being made for the Upgrade are assessed for their applicability for a future more considerable extension. For example, several devices emitting and receiving acoustic signals will be installed to explore sub-cm geometry calibration techniques and potential acoustic detection of neutrino interactions in the ice. Additionally, promising novel in-ice optical module designs will be included in small quantity R&D (“special devices”) deployments on the Upgrade strings. These new detector elements include the IceCube-Gen2 Optical module, which combines elements of the IceCube Upgrade optical sensors, but elongated to fit into a smaller diameter cylindrical or egg-shaped housing that could dramatically reduce drilling costs. Other R&D modules are the WOM (wavelength-shifting optical module), a revolutionary step in gaining effective collecting area without increased photocathode size and price, the FOM (fibre optical module), a similar cost-saving strategy that deploys fibres into the drill hole.
What’s next?
The IceCube Upgrade project will significantly increase IceCube’s sensitivity to lower-energy neutrinos and improve the fidelity of all past and future data. In addition, it is a step in the development of IceCube-Gen2, a much larger extension of the IceCube Neutrino Observatory that will increase the in-ice instrumented volume by a factor of ten, paving the way to new discoveries with cosmic neutrinos.
References
- Fukuda et al. (Super-Kamiokande), Phys. Rev. Lett. 81, 1562 (1998), hep-ex/9807003.
- Science 378, 6619, 538-543 (2022)
- Nakahata (Super-Kamiokande), PoS NEUTEL2015, 009 (2015).
- G. Aartsen et al. (IceCube), Phys. Rev. D91, 072004 (2015), 1410.7227.
- Stuttard et al. (IceCube), PoS NuFACT 2019, 099 (2019).
- G. Aartsen et al. (IceCube), Phys. Rev. D 99, 032007 (2019).
- G. Aartsen et al., J. Phys. G 48, 060501 (2021), Astro-ph.HE/2008.04323.