EPRI and Vanderbilt University highlight the importance of incorporating risk insights early in the advanced nuclear reactor design process to shorten schedules and enhance safety.
Increased understanding of the impacts of greenhouse-gas-emitting forms of electric power production on climate change has led to industry, academic, and government-sponsored research into viable alternatives. The majority of experts agree that no one technology can solve the grand challenge of decarbonising power production and that the most viable future energy portfolios include a variety of renewable and zero carbon emission energy sources.2 Advanced reactors are nuclear reactor concepts that utilise novel fuel forms, coolant options, or operational concepts that are intended to exhibit ‘significant improvements’3 over the present generation of light water reactors. Such reactors can play an important role in planned zero carbon energy portfolios since they can be used as a source of baseload energy to supplement intermittently available renewable energy sources, such as wind and solar.
However, a number of external factors have the potential to impact the viability and deployment of advanced nuclear reactors. These factors include:
- Varying levels of public acceptance for nuclear energy due to perceptions regarding safety and waste disposal;
- The capabilities of existing regulatory frameworks to assess and verify expectations regarding the ‘enhanced safety’ that is expected for these reactors, which look and operate differently than current reactors; and
- The infrequent application of industry-standard nuclear safety analysis methods at the early stages of advanced reactor design to yield information that can identify hazards and preventive/mitigative design features.
Methodologies for integrating safety analysis early in the advanced nuclear reactor design process
For the reasons stated above, researchers at Vanderbilt University and the Electric Power Research Institute (EPRI) have partnered to investigate ways to integrate safety analysis early in the advanced nuclear reactor design process. The goal of this research is to understand reactor safety better and identify design insights for improving safety in ways that meet regulatory expectations, and help inform later-stage quantitative analyses in ways that are implementable by design organisations.
Through this research, EPRI and Vanderbilt have identified hazard evaluation tools and procedures that often originated within other industries, such as the chemical process and aerospace industries, but can be tailored to analyse early-stage advanced nuclear reactor designs. These techniques, many of which fall under the umbrella of process hazards analyses (PHAs),4 can be applied iteratively as part of advanced reactor design through a process described as ‘Safety in Design’. The application of these techniques can also be tailored to the degree of design maturity at the time of analysis.
To get the most value out of the application of PHA methods during design, it is important to know which methods to choose. Vanderbilt and EPRI have used existing guidance from the U.S. Nuclear Regulatory Commission (NRC) for fuel cycle facilities5 to help identify which PHA methods, such as hazard and operability studies (HAZOP) and failure modes and effects analysis (FMEA), should be applied at various stages of design maturity to provide the most value to a design project. These PHA techniques are recognised in advanced nuclear reactor design and safety-analysis-related technical guidance documents and standards, such as the Nuclear Energy Institute’s Risk-Informed Performance-Based Technology Inclusive Guidance for Advanced Reactor Licensing Basis Development, NEI 18-04 (which has been endorsed by the NRC in Regulatory Guide 1.233)6 and the American Society of Mechanical Engineers and American Nuclear Society in their Non-LWR Probabilistic Risk Assessment (PRA) Standard.7
Not only have EPRI and Vanderbilt theorised the possibility of using SiD and PHA methods to influence advanced reactor design, but the team has also put these methodologies into practice. They have worked with advanced nuclear reactor designers to apply the methodology to six reactor designs at various stages of design maturity – designs that employ differing core layouts, moderators, coolants, and neutron energy spectrums. In addition to these real-world SiD applications – providing advanced reactor designers with usable insights at the time of the analysis – these evolutions provide training to the designers on how and when to employ SiD methods as their project progresses. For example, Vanderbilt and EPRI’s research has identified guidance on the types of PHA methods that are most suited to provide value added to a design project when applied at the pre-conceptual, conceptual, and preliminary stages of design as shown in Fig. 2.8
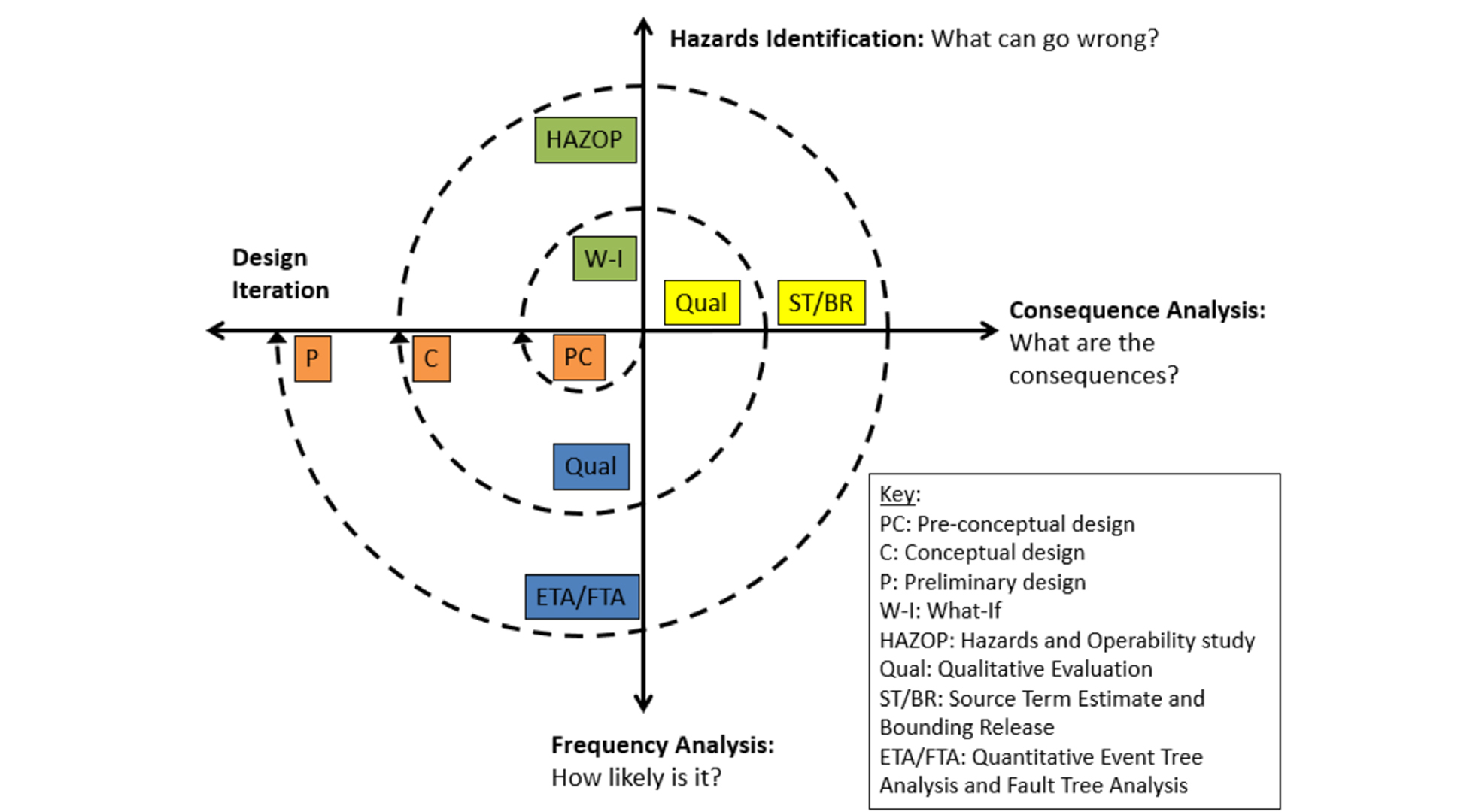
To date, many of the PHAs that EPRI and Vanderbilt have performed have been for molten salt reactor (MSR) subsystems. MSRs are particularly susceptible to challenges in nuclear safety analysis and licensing frameworks since their operational concept, in which molten salt can be used as both fuel and coolant, starkly contrast with existing light-water reactors (LWRs) and other advanced reactor concepts. Unlike LWRs, a comprehensive list of accident scenarios that need to be considered for MSRs during the reactor’s safety analysis is still to be developed. EPRI and Vanderbilt’s detailed application of SiD on MSR subsystems and test platforms has, in addition to being used to gain design insights, been used to systematically identify accident scenarios for MSRs and MSR subsystems.9
The results from these evaluations can be used to feed into later-stage quantitative safety analyses that continue to build the safety case for the design. These quantitative safety evaluations, often called probabilistic risk assessments (PRAs) or probabilistic safety assessments (PSAs), are expected to be a critical element of the safety case presented to regulators. As shown in Fig. 3, EPRI and Vanderbilt’s research has demonstrated that SiD methods can allow designers to incrementally develop the building blocks of a PRA/PSA with documented ties to component reliability, safety analysis data, and the engineering design baseline. In addition to supporting later-stage safety analyses, EPRI and Vanderbilt’s application of SiD methods to these real-world problems has helped reactor designers by demonstrating the direct incorporation of safety into the design process; identifying potential operability issues at an early stage for design attention; and helping to identify and prioritise needed research and development.
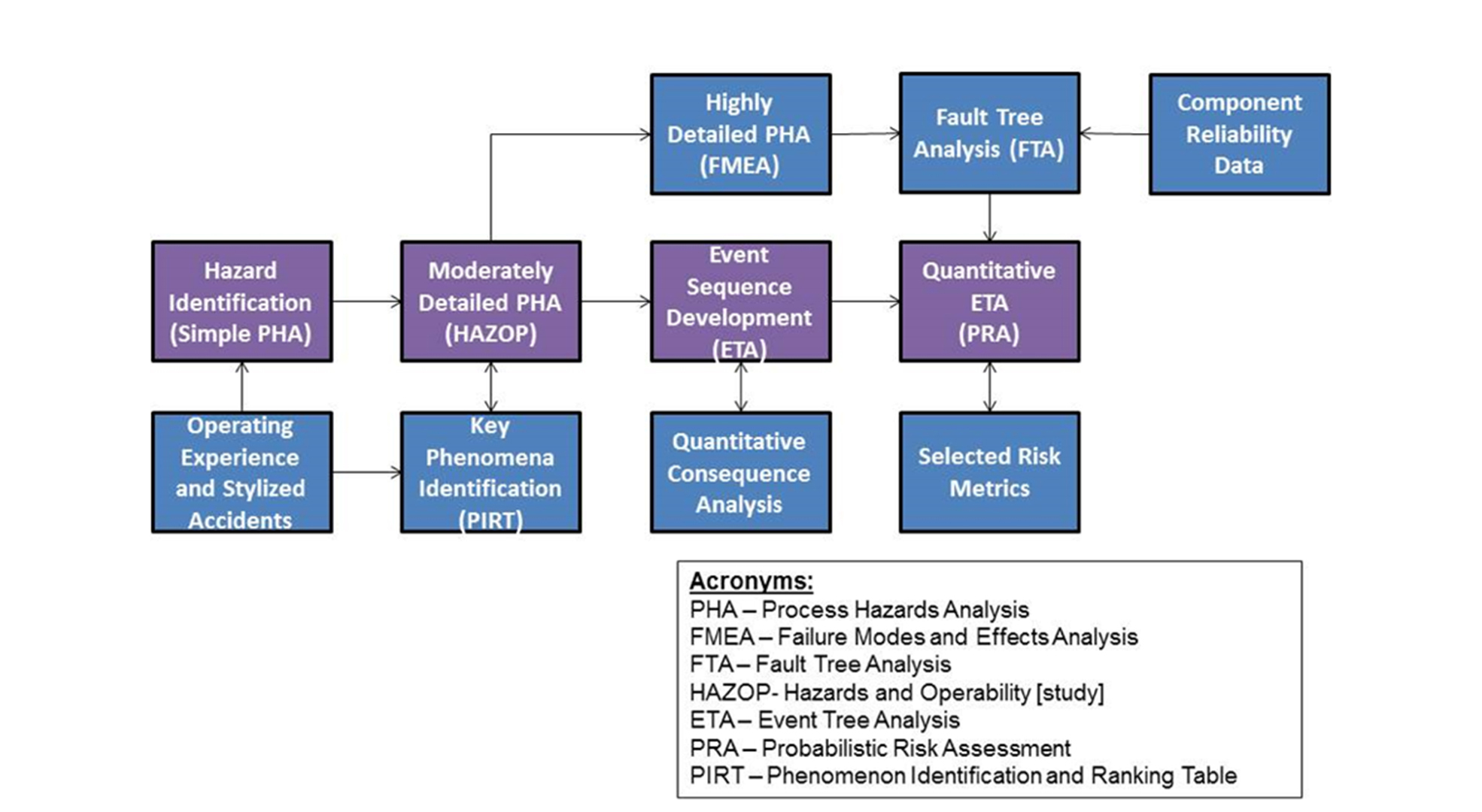
Analysing historical reactor designs to yield insights for modern reactor designers
Vanderbilt and EPRI have also used the SiD methodology to analyse some historical examples of advanced nuclear reactor designs. One such system, shown in Fig. 1, is the Molten Salt Reactor Experiment (MSRE)– the last MSR ever operated (and one of only two). They have evaluated five of the unique subsystems and components within the MSRE using methods such as FMEA and HAZOP.10 The unique subsystems analysed within the MSRE include the freeze valve, an alternative to traditional valves being implemented in modern MSR designs, and the off-gas system – a critical system within MSRs used to contain and treat the fission gases that evolve from the fuel salt.
The MSRE shut down in December of 1969; however, these SiD evaluations of historical MSRE subsystems can still provide fresh design insights for modern-day MSR designers that help to bridge the knowledge gap that exists because of the over 50-year time period since the last MSR operated. These evaluations of the MSRE have helped to identify weaknesses of past designs to be avoided in future designs, offer insights on potential solutions for current design issues, and identify hazards/operational deficits for the design elements which were evaluated that may not be immediately obvious at the level of design maturity that current projects have reached. As an example, one of Vanderbilt’s recent journal articles on the subject that documents the SiD analysis of the freeze valve subsystem11 has been read more than 250 times in the less than two years since it was published.
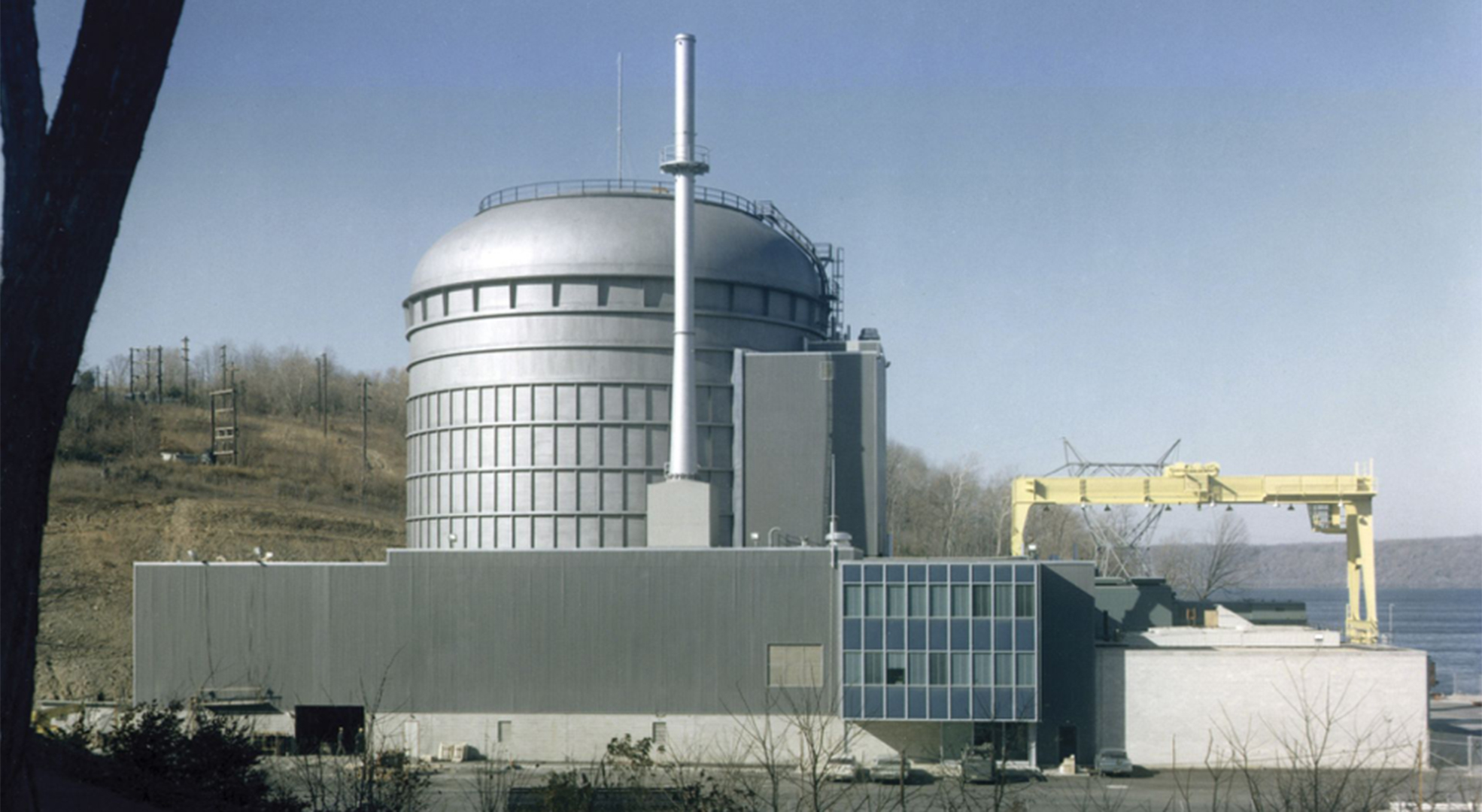
Future plans for development and growth
Going forward, EPRI and Vanderbilt have no plans to slow down. They have already started work with additional advanced nuclear reactor designers developing concepts other than MSRs (such as modern evolutions of the high-temperature gas reactor concept [HTGR] shown in Fig. 4) to integrate SiD methods into their design processes. Vanderbilt is also working on a U.S. Department of Energy, Office of Nuclear Energy-funded project to develop a molten salt sampling system for use within future MSRs12 and is using the SiD methodology to develop this important MSR subsystem. EPRI and Vanderbilt are also working to incorporate PHA tools and SiD methodologies into cutting-edge, model-based systems engineering approaches for nuclear design projects, with the potential for expanding this work to support commercial modular fusion power systems.
The Vanderbilt and EPRI team’s work can be viewed at:
- Program on Technology Innovation: Early Integration of Safety Assessment into Advanced Reactor Design—Project Capstone Report. EPRI, Palo Alto, CA: October 31, 2019. Report 3002015752.
- Molten Salt Reactor Experiment (MSRE) Case Study Using Risk-Informed, Performance-Based Technical Guidance to Inform Future Licensing for Advanced Non-Light Water Reactors, Southern Company and EPRI, 2019. Report EPRI AR LR 2019-06. (Can be found on the NRC website under ADAMS accession number ML19249B632)
- B M Chisholm, S L Krahn, K N Fleming, A systematic approach to identify initiating events and its relationship to Probabilistic Risk Assessment: Demonstrated on the Molten Salt Reactor Experiment, Progress in Nuclear Energy, 129:103507 (2020).7
References
- M W Rosenthal, An Account of Oak Ridge National Laboratory’s Thirteen Research Reactors Rev. to March 2010, ORNL/TM-2009/181, Oak Ridge National Laboratory, 2010
- Rogelj, J , D Shindell, K Jiang, S Fifita, P Forster, V Ginzburg, C Handa, H Kheshgi, S Kobayashi, E Kriegler, L Mundaca, R Séférian, and M V Vilariño, 2018: Mitigation Pathways Compatible with 1.5°C in the Context of Sustainable Development. In: Global Warming of 1.5°C. An IPCC Special Report on the impacts of global warming of 1.5°C above pre-industrial levels and related global greenhouse gas emission pathways, in the context of strengthening the global response to the threat of climate change, sustainable development, and efforts to eradicate poverty [Masson-Delmotte, V, P Zhai, H-O. Pörtner, D Roberts, J Skea, P R Shukla, A Pirani, W Moufouma-Okia, C Péan, R Pidcock, S Connors, J B R Matthews, Y Chen, X Zhou, M I Gomis, E Lonnoy, T Maycock, M Tignor, and T Waterfield (eds)]. In Press
- Nuclear Energy Innovation Capabilities Act of 2017, U.S. Public Law. 115-248 (2018)
- Guidelines for Hazard Evaluation Procedures. 3rd ed. New York, N.Y.: CCPS, Center for Chemical Process Safety, 2008
- U.S. Nuclear Regulatory Commission, Standard Review Plan for Fuel Cycle Facilities License Applications, NUREG-1520 Rev. 2, 2015
- Nuclear Energy Institute (NEI), Modernisation of Technical Requirements for Licensing of Advanced Non-Light Water Reactors: Risk-Informed Performance-Based Technology Inclusive Guidance for Non-Light Water Reactor Licensing Basis Development, Rev. 1, NEI 18-04, Washington, DC (2019)
- American Society of Mechanical Engineers (ASME), Probabilistic Risk Assessment Standard for Advanced Non-Light Water Reactor Nuclear Power Plants, ANSI/ASME/ANS RA-S-1.4-2021 (2021)
- Program on Technology Innovation: Early Integration of Safety Assessment into Advanced Reactor Design—Project Capstone Report. EPRI, Palo Alto, CA: October 31, 2019. Report 3002015752.
- B M Chisholm, S L Krahn, K N Fleming, A systematic approach to identify initiating events and its relationship to Probabilistic Risk Assessment: Demonstrated on the Molten Salt Reactor Experiment, Progress in Nuclear Energy, 129:103507 (2020)
- Molten Salt Reactor Experiment (MSRE) Case Study Using Risk-Informed, Performance-Based Technical Guidance to Inform Future Licensing for Advanced Non-Light Water Reactors, Southern Company and EPRI, 2019. Report EPRI AR LR 2019-06. (Can be found on the NRC website under ADAMS accession number ML19249B632)
- B M Chisholm, S L Krahn, A G Sowder, A unique molten salt reactor feature – The freeze valve system: Design, operating experience, and reliability, Nuclear Engineering and Design, 368 (2020)
- M Harkema, P Marotta, S Krahn, “Fuel Salt Sampling and Enriching Technology Design Development – Project Update,” Transactions of the American Nuclear Society, 124(1): 465-467, 2021
- P Samanta, D Diamond, W Horak, Regulatory history of non-light-water reactors in the U.S., Nuclear News, 2020