The CMS upgrades to the Tracker for the Large Hadron Collider operations and their potential are described here.
The CMS collaboration at the CERN LHC is preparing a major upgrade of the detector in preparation for the High-Luminosity LHC operations starting in 2029. Following the discovery, in 2012, of the Higgs boson, the experiments at the CERN Large Hadron Collider have continued to study the Higgs boson and measure its properties.
The Higgs boson plays a unique role in the standard model of particle physics. The Higgs field is responsible for the mass of the fundamental particles through their coupling to the Higgs field. The stronger the coupling, the more massive the particles. One beautiful way to show this is to measure the coupling strength of particles to the Higgs and plot the coupling versus the mass of the particle. This is shown in Fig. 1, where we can see good agreement between the coupling strength and mass over many orders of magnitude. However, the precision of many of these measurements is limited, and to provide a stricter test of the agreement with the standard model, significantly more data is required.
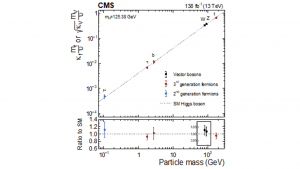
Hence, an upgrade for the High-Luminosity LHC (HL-LHC), has been undertaken to increase the luminosity of the LHC by about an order of magnitude. This will allow much more precise tests of the standard model Higgs mechanism. In addition to Higgs studies, the HL-LHC upgrades will also improve the sensitivity in many searches for new physics beyond the standard model.
HL-LHC upgrades
The HL-LHC upgrades will enable the collection of a data set that is an order of magnitude larger than during the pre-HL-LHC operation ending in 2026. This is enabled using stronger focusing of the beams at the interaction points. Each bunch collision, every 25 nanoseconds, will produce an average of up to 200 proton-proton interactions. After Run 3 ends in 2026, these upgrades to the Large Hadron Collider will be installed with the start of HL-LHC data taking in 2029.
The experiments at the LHC will need to be upgraded to handle the significantly higher data rate at the HL-LHC. There are three primary challenges:
- The higher luminosity will increase the data rates from the detector. We need to have sufficient bandwidth to read out the data and sufficiently fine granularity in the detectors to be able to separate overlapping objects;
- The detectors need to survive in the harsh radiation environment at the HL-LHC for an integrated luminosity of up to 4000fb1. This is particularly a challenge for the detectors near the interaction point and in the very forward region of the detector; and
- The online event selection, known as triggering, becomes much more challenging with the higher data rate. We need to continue maintaining trigger thresholds low enough to efficiently trigger on electroweak scale physics, W, Z, H, and top, while maintaining a sufficiently low trigger rate such that the data can be processed and recorded.
To meet these challenges, the CMS (Compact Muon Solenoid) experiment has prepared a set of upgrades documented in the Technical Proposal.2 We describe here the upgrades to the CMS tracking detectors, the Inner Tracker consisting of a pixel detector and the Outer Tracker consisting of Strip and Macro Pixel detectors. The Outer Tracker is built from a new type of module that can discriminate against hits from low momentum particles and provide input to the new Track Trigger that will be part of the upgrades.
Outer tracker and track trigger
The upgrade of the CMS tracker consists of a complete replacement of the Outer Tracker to install a new detector that can handle the challenging environment at the HL-LHC:
- More radiation-hard to withstand the harsh radiation environment;
- Larger readout bandwidth to handle the higher data rate; and
- Higher granularity to resolve the higher density of particles produced.
In addition to these improvements over the current detector, the upgraded tracker is specifically designed to provide charged particle tracking information to the level one trigger. This is a new capability for the CMS experiment and requires a very different approach to the tracking detector implementation.
For the implementation of charged particle tracking at level one (L1), the hardware trigger, we need access to charged particle hit information for each proton-proton bunch crossing every 25 nanoseconds. With the readout technology available today, we can’t read out all hits from the tracker on each bunch crossing. To provide tracking information for each bunch crossing, a novel module consisting of two parallel silicon sensors is used, see Fig. 2. The readout electronics look for correlations between hits in the two sensors. Charged particle trajectories bend in the CMS 3.8T magnetic field, and low momentum particles produce hits that are separated between the two sensors. By selecting pairs of hits, or stubs, that are in a narrow window of each other in the two sensors, we can select hits from particles with a high transverse momentum and reduce the data volume for trigger readout by an order of magnitude or more. This allows readout of trigger primitives for each bunch crossing at 40MHz using a single ten Gigabits per second (Gbits/s) optical link for each detector module.
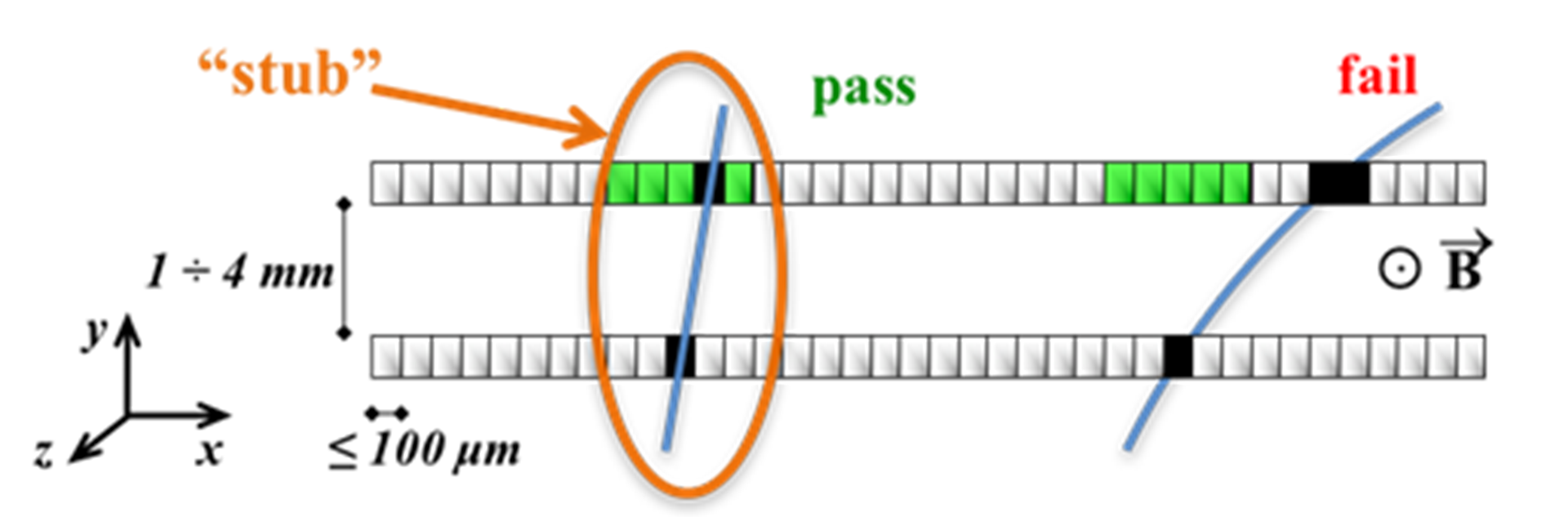
The upgraded detector will be constructed from two types of modules, see Fig. 3. At distances close to the beamline, less than about 50cm, we use modules that consist of one strip sensor and one macro pixel sensor (PS modules). The macro pixels are about 100µm × 1.5mm, and the strips are 2.5cm long. The higher granularity at the smaller radii is required to handle the higher data rate near the interaction point. The macro pixels also provide accurate longitudinal measurements along the beamline, which is important for separating charged particles from different proton-proton interactions. At larger radii, modules consisting of two strip sensors, or 2S modules, are used. The layout of the tracker detector is shown in Fig. 4. The outer tracker consists of six-barrel layers and five disks on each side of the interaction point. The whole detector consists of about 13,300 modules. The modules are tilted in the three innermost barrel layers such that the normal points towards the interaction point. This helps reduce the amount of material a particle will traverse as it propagates through the tracking detector.
The stubs from these modules are processed by the track-finding system. The track trigger is a new capability for the CMS HL-LHC upgrades.3 In the L1 trigger, only information from the muon detectors and the calorimeters are available in the current detector. The aggregate bandwidth into the track-finding system is about 40 Terabits per second, and about 15,000 stubs are received in each bunch crossing every 25 nanoseconds. The goal of the track finding is to reconstruct the charged particle trajectories with transverse momentum above two gigaelectronvolts (GeV). There are about 200 such charged particles in average per bunch crossing, or about 8 × 109 per second.5
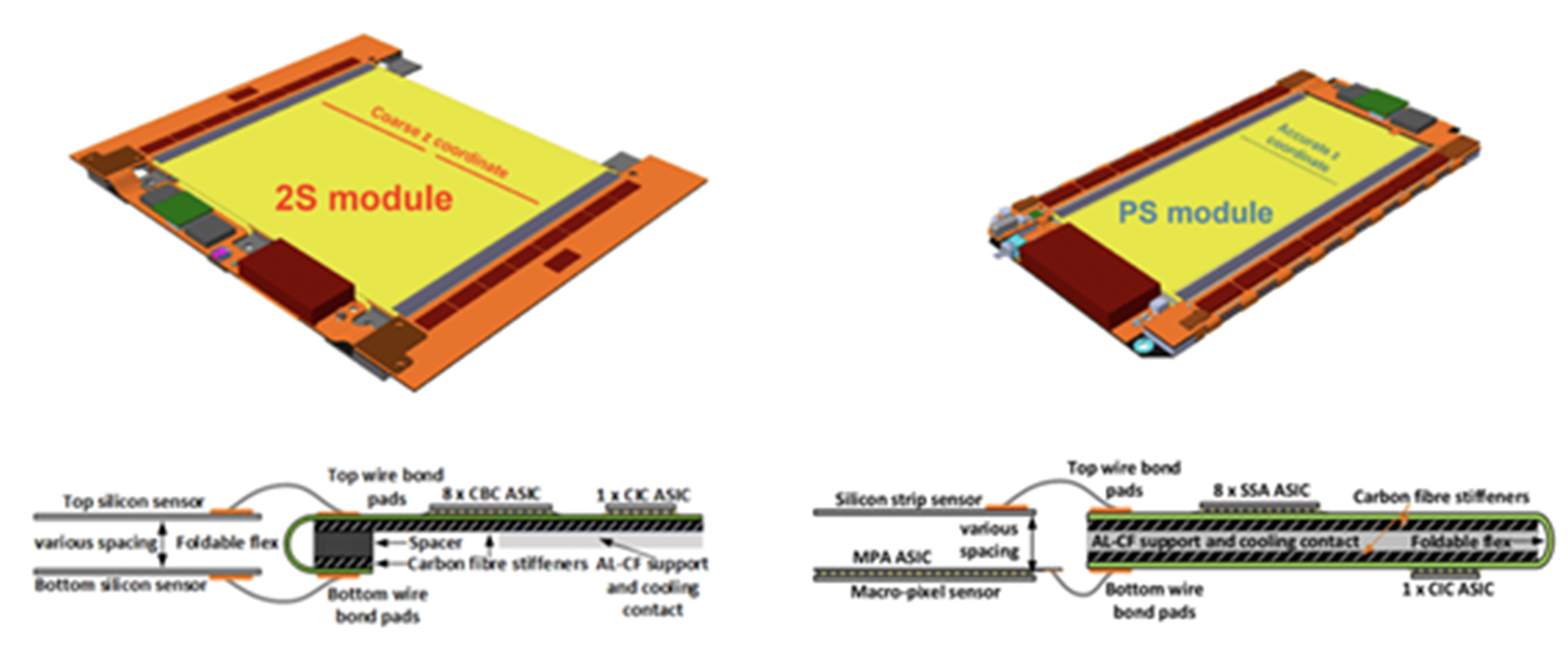
The implementation of the charge particle reconstruction uses powerful Field Programmable Gate Arrays (FPGAs) on custom-designed boards, see Fig. 5. These boards use high-speed optical links operating at 25 Gbits/s to bring data in and out from the board with low latency. The FPGAs (Xilinx VU13P) allow I/O at 25 Gbits/s per serial link, matching the optical links and providing powerful processing for the implementation of the track-finding algorithm. The track-finding is implemented using 162 track-finder boards and utilises both spatial and temporal multiplexing. The detector is divided into nine sectors and 18 time-multiplexing slices. The track finding implements a ‘road search’ algorithm using seeds formed in nearby layers or disks. These seed trajectories are then projected to other layers or disks in the detector to find matching stubs and form tracks. These track candidates are fit using a Kalman filter to find improved track trajectory parameters.
Inner Tracker forward pixel detector
For the Inner Tracker upgrade, where the occupancies are very high, CMS uses a silicon pixel detector with a pixel size of 25 × 100µm2. This pixel size is smaller by a factor of six than the current pixel detector. Fig. 4 shows the layout of the pixel detector as the innermost tracking elements in yellow and green.
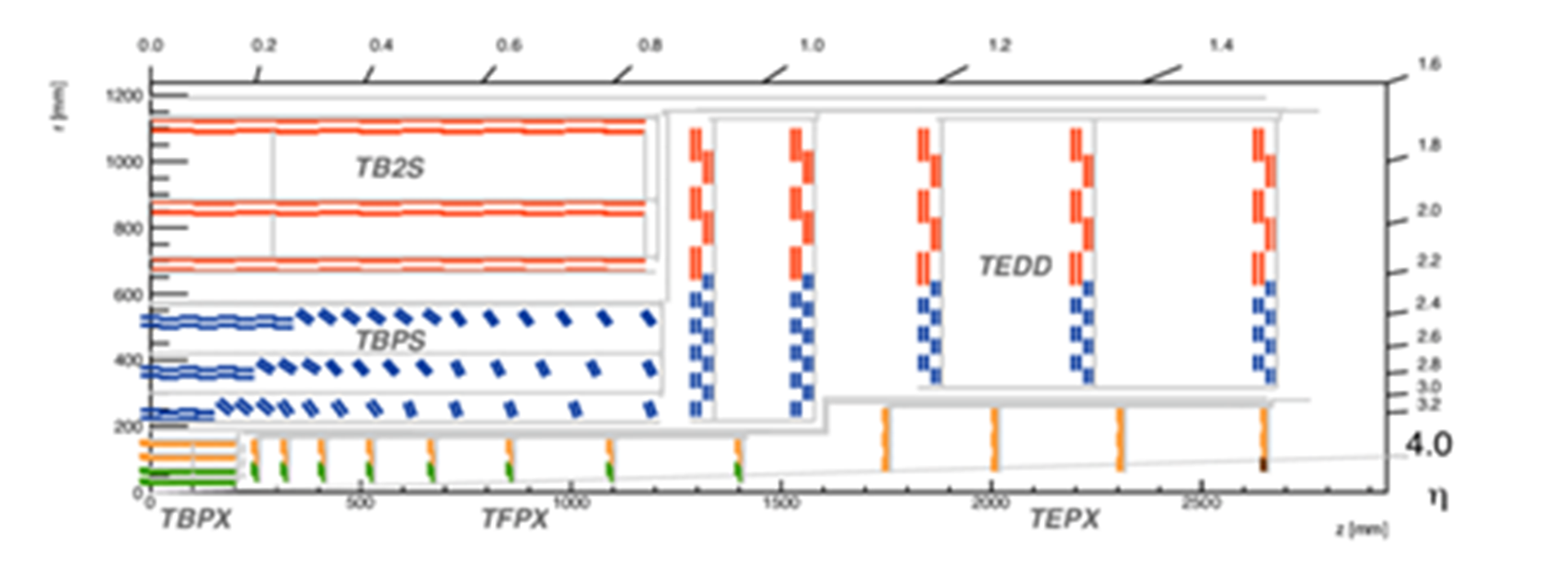
The upgraded pixel detector will have 8+4 disks on each side of the interaction point compared to the three disks in the current detector. This extends the forward tracking coverage from about | ŋ | = 2.5 in the current detector to | ŋ |= 4.0, corresponding to an angle of about two degrees from the beamline. A CAD image of the upgraded CMS pixel detector is shown in Fig. 6.
The pixel detector is closest to the interaction point and sees the highest particle fluences. The innermost pixel sensors see a fluence of over 1016 neutron equivalent per cm2. The sensors and readout chip have been carefully designed to ensure they will survive in this harsh radiation environment. The sensors are designed to be thin, 150um, and operated with a high bias voltage, up to 800V, in order to remain efficient after the radiation exposure. Furthermore, the detectors will be operated cold, at a temperature around -30˚C, to reduce the damage from radiation. This is challenging as the electronics in the pixel detector generate about 40kW, and we need to keep the mass to a minimum. To provide efficient cooling with low mass, we use a two-phase CO2 cooling system and mechanical structures made from titanium, carbon fibre, and carbon foam. The detector modules are mounted on Dee structures and assembled into cartridges which are independent detector units that can be installed and removed from the detector. For the TFPX system, assembled in the US, there are 32 cartridges.
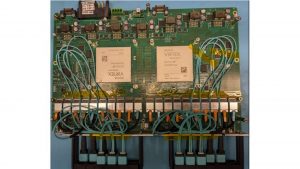
We have now built prototypes of all different components, detector modules, mechanical structures, and electronics, and we are now proceeding towards the production phase of the project with the goal of having the detector components ready by the middle of 2027. Installation and integration with the rest of the CMS detector will be done in 2028.
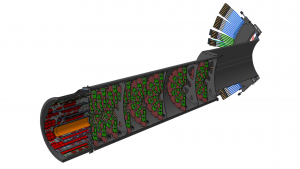
Summary
The CMS collaboration is pursuing an exciting upgrade of the CMS detector for the Large Hadron Collider. There are many novel detector concepts that will provide completely new handles during the HL-LHC operation. In this article, I highlighted two of these: the tracker with the track trigger and the new extended pixel tracker.
Acknowledgement
This work was supported by NSF-PHY-2209443 and NSF-PHY-1946735. I thank all my collaborators on CMS and the staff at CLASSE.
References
- CMS Collaboration. A portrait of the Higgs boson by the CMS experiment ten years after the discovery. Nature, Vol 607, 60 (2022)
- CMS Collaboration. Technical Proposal for the Phase-2 Upgrade of the CMS Detector. Tech. Rep. CERN-LHCC-2015-010, CERN (2015)
- A Ryd and L Skinnari, Tracking Triggers for the HL-LHC, Ann. Rev. Nucl. Part. Sci. 70, 171-195 (2020)
- CMS Collaboration. The Phase-2 Upgrade of the CMS Tracker. Tech. Rep. CERN-LHCC-2017-009, CERN (2017)
- CMS Collaboration. The Phase-2 Upgrade of the CMS Level-1 Trigger. Tech. Rep. CERN-LHCC-2020-004, CERN (2020)
Please note, this article will also appear in the sixteenth edition of our quarterly publication.