Jennifer Littlejohns, Program Director of the Advanced Clean Energy program at the Energy, Mining and Environment Research Centre, discusses the direction of the program, which aims to support Canada’s emerging battery supply chain.
For Canada to meet its 40-45% emission reduction targets in 2030 and achieve net zero emissions by 2050, focused research is required to develop clean energy technologies and bring them from the lab to the market. The National Research Council of Canada (NRC) conducts battery research and development along the entire supply chain, from mining to end-use integration.
The NRC’s Advanced Clean Energy program was developed as part of the Energy, Mining and Environment Research Centre to host strategic research and development (R&D) projects. With the aim to support the transition to a low carbon economy, these projects take into account the priorities of the Government of Canada and Canadian industry.
To facilitate the transition to electrification in many sectors in Canada, a key pillar of this strategic program is to foster Canada’s energy storage and battery supply chain, including the development and recovery of minerals and materials, for stationary and motive applications.
While the program was launched in 2021, it builds on battery development research capabilities that have been growing for decades at the NRC. The program hosts more than 25 projects in the battery energy storage pillar for, and in collaboration with, industry, academic, and government partners, both nationally and internationally.
Research is conducted at sites across Canada, including: Boucherville, Quebec, with a focus on mineral sensing and processing; Ottawa, Ontario, with activities including recycling, new battery chemistries, solid-state electrolytes, and safety; Mississauga, Ontario, looking at new battery materials and discovery; and, Vancouver, British Columbia, with research in areas of redox flow and state of charge, state of health, and standards development.
There are many unique facilities at the NRC that are used to conduct these strategic projects, including the battery performance and safety evaluation facility, small battery development and testing facilities, and the battery recycling pilot plant.
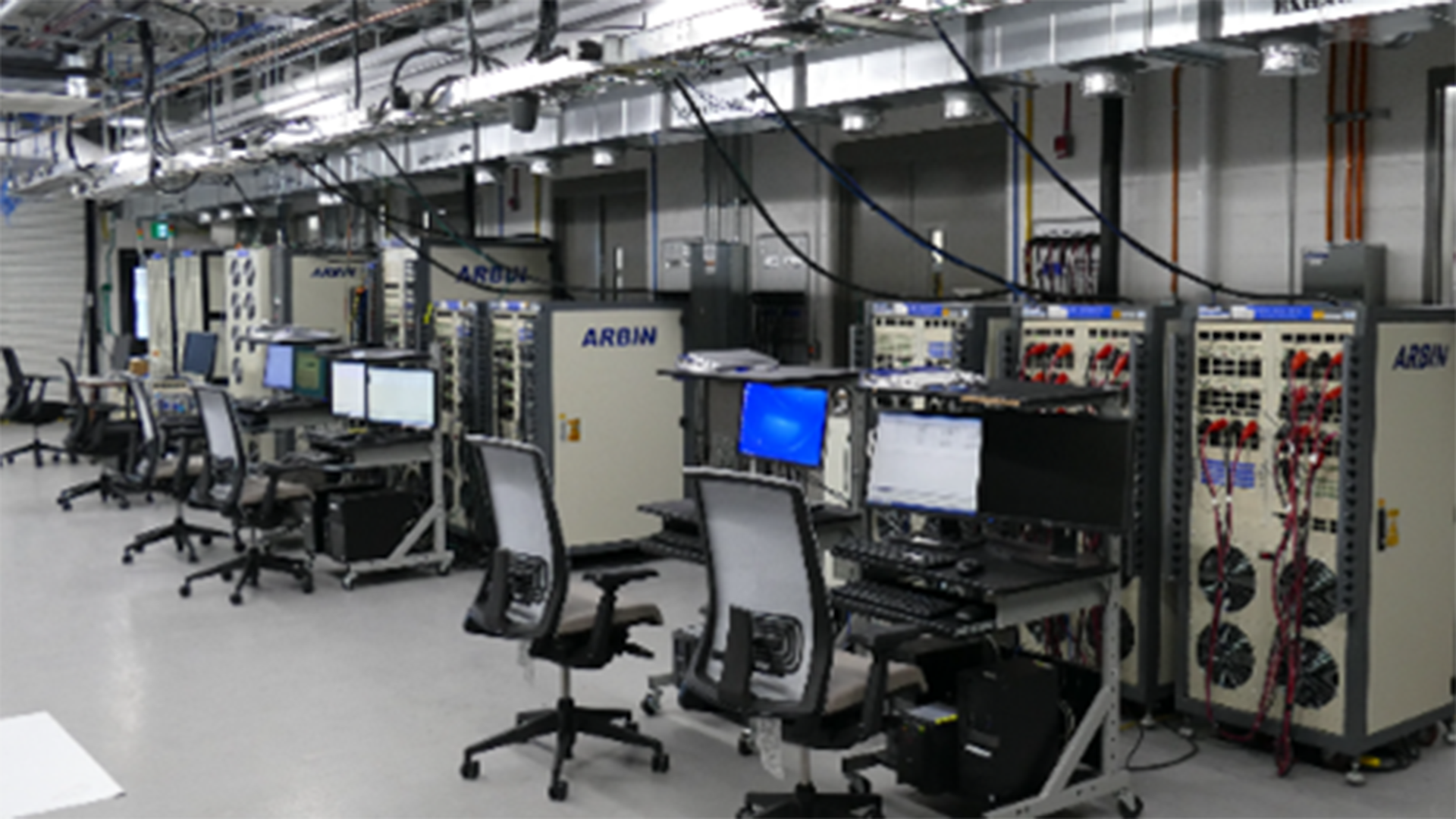
The aims of the Advanced Clean Energy program
The program aims to develop a local, ethical, and clean battery supply chain as a major opportunity for the Canadian market.1 Four key areas of research are being conducted to support an environmental, social, and governance (ESG) focused supply chain:
Battery materials sensing and recovery
Detection and quantitative analysis of Canadian-specific materials such as lithium, lithium brine, nickel and cobalt salts, vanadium, and natural flake graphite in co-production/processing for use in battery anodes and cathodes of sensing technologies for lithium-ion batteries (LIBs).
Battery materials and components
Evaluation and improvement of battery properties such as capacity retention, cyclic stability, specific capacity and current density, and rate performance for various chemistries such as wet lithium cells, flow batteries, and next-generation solid-state batteries. Development of new membranes/separators, investigation of cathode-electrolyte interfaces, and battery management systems.
Battery materials recycling and end of life
The analysis, development, and piloting of recycling processes, as well as diagnostics for the state of health of consumer and electric vehicle (EV) batteries, to determine if the cell should be reused in alternate applications such as stationary storage or backup, remanufactured into a new pack or module, or sent for large-scale recycling.
Battery safety and performance
Modelling and experimentation to identify high-risk failure scenarios for testing the safety of lithium-ion cells, thermal abuse testing and early detection for LIBs’ thermal runaway based on gas sensing, and experimental studies on suppression of fire by inert gas, amongst others.
Below, the new projects for Canada’s critical minerals initiative will be described in more detail.
Toward a North American LCA-based certification for critical minerals
In addition to the technical and economic challenges of material sourcing for use in LIBs, there is a growing interest in quantifying the sustainability of critical minerals and battery component supply chains. Life cycle analysis (LCA) can be a key tool in providing such assessments, identifying hot spots to reduce negative impacts, while guiding decision making by end users, such as EV manufacturers and consumers.
Successful LCA depends on the quality of underlying life cycle inventory (LCI) data surrounding the extraction and downstream processes for raw materials. Thus, to increase confidence in LCA results, the construction of a harmonised and transparent methodology is desirable.
The NRC is adapting an LCA framework to address this challenge in the context of critical battery minerals,2 leveraging publicly available information and process modelling to estimate LCI data and environmental impacts in the context of Canada’s battery supply chain.
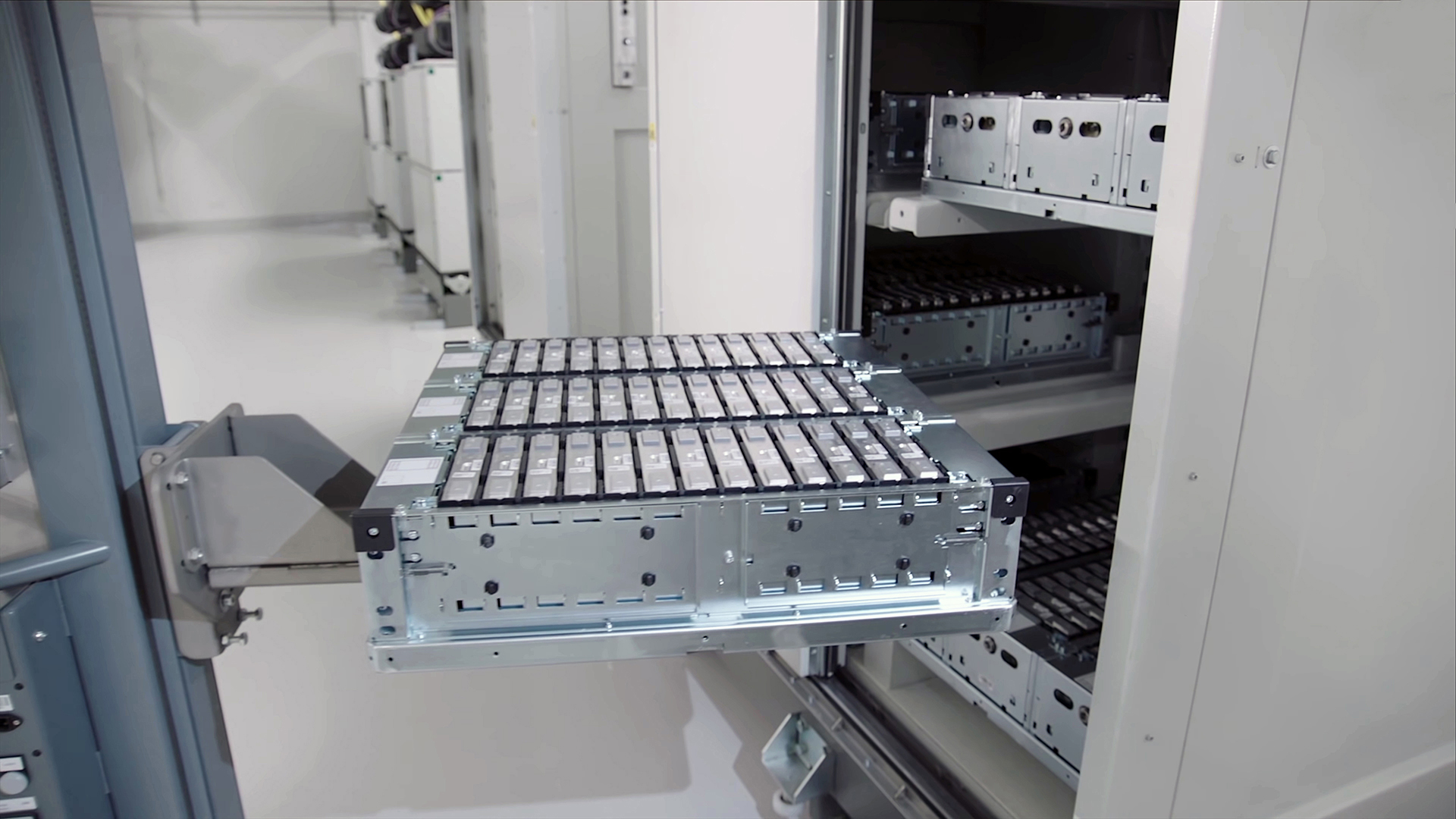
LIB recycling: Scale-up supported liquid membrane (SLM) piloting
Over the next decade, the demand for LIBs for use in EVs is projected to increase substantially. Concurrently, greater quantities of spent LIBs will require sustainable methods for disposal. Devising an effective recycling strategy for LIBs, including processing and policymaking, is an essential step towards developing a circular economy model, thus avoiding landfilling and providing an alternative supply of energy metals.
Currently, spent LIBs are manually disassembled, shredded, and physically separated. Plastics, steel, copper, and aluminium are returned to current processing chains for recycling. The cathode and anode materials end up in black mass from which only copper (Co) and nickel (Ni) are typically recovered through smelting. These metals do not necessarily return to battery manufacturing. Recent LIB recycling, which adopts hydrometallurgical approaches, produces salts of manganese (Mn), Co, and Ni along with lithium hydroxide/carbonates, that are then returned for LIB manufacturing.
Solvent extraction, using conventional mixer-settlers, is typically used and requires a large volume of organic extractant. The NRC is developing supported liquid membrane (SLM) technologies that would consume a fraction of the organic extractant required in a conventional system. To date, bench-scale testwork on synthetic solutions has demonstrated successful Co-Ni separation where 100% Co extraction was achieved while recovering only 200 ppm of Ni from a feed of 10,000 pm Ni.
As current work continues with the development of Mn-Co-Ni separation, and demonstration of using hollow fibre SLM in batch testing, an integrated pilot plant to simultaneously separate Mn-Co-Ni, using up to ten modules, is at the planning stage.
The envisioned pilot plant will process pregnant leach solution generated from two tonnes of ‘real’ black mass. Commissioning and operation are expected in 2023.
Direct lithium extraction from oilfield brines
Canada’s natural resources include many of the essential minerals identified by Natural Resources Canada.3 This includes lithium, a key element to LIBs, which can be found in two forms, hard rock and oilfield brine.
Oilfield brines typically contain a low content (less than 100 ppm) of lithium and a high concentration of impurities such as sodium (Na), potassium (K), magnesium (Mg), calcium (Ca), and strontium (Sr). Existing industrial extraction technology is difficult and uneconomical to process this type of low-grade brine. The objective of this research study at the NRC is to explore a green and promising lithium-ion exchange technology for lithium extraction from the low grade Li-containing brines. This study covers two technology streams: electrochemically assisted lithium-ion sieve technology (e-LIS), and lithium-specific extractant development to be used with SLM.
Quantifying precursors and intermediates of nickel and iron for potential use in battery material
According to the United States Geological Survey (USGS), over 55% of the world’s mined nickel is targeted for the stainless steel industry, with only 10% for chemical products. This has created an intermediate bottleneck in the supply chain for EV adoption, as most LIBs preferred in North America are high nickel content cathodes. No immediate relief is in sight, as most mines take 15-20 years to commercialise. Cobalt presents a further challenge in Canada’s battery supply chain due to the associated detrimental socioeconomic impact of mining and refining.
The NRC is exploring an integrated approach whereby new cathode chemistry based on nickel–iron (Ni-Fe) is developed and derived from converted nickel pig iron (NPI) or ferronickel into an intermediate product for cathode precursors. The processing investigation will evaluate selective leaching of Ni with some Fe or will look to leach the Ni and Fe together to use as a precursor to synthesise the cathode. Cathode development will look at novel Ni-Fe-based cathodes with the emphasis on impurity tolerance, which may subsequently reduce processing requirements. This holistic approach will maximise feedstock limitation, processing intensity for purity, and battery material supplies.
References
1 publications.gc.ca/collections/collection_2021/rncan-nrcan/M4-203-2020-eng.pdf
2 doi.org/10.1016/j.jclepro.2021.128394
3 www.nrcan.gc.ca/our-natural-resources/minerals-mining/critical-minerals/23414
Jennifer Littlejohns
Program Director
Advanced Clean Energy
National Research Council Canada
https://nrc.canada.ca/en
https://www.linkedin.com/in/jennifer-littlejohns-48768911/
Please note, this article will also appear in the tenth edition of our quarterly publication.