ELI-NP implements a unique research infrastructure dedicated to Nuclear Photonics, hosting the most powerful lasers in the world.
Extreme Light Infrastructure – Nuclear Physics (ELI-NP) is a new research infrastructure currently under implementation in Romania by the National Institute for Research and Development in Physics and Nuclear Engineering – Horia Hulubei. ELI-NP is located on the Magurele Physics Platform in the southern part of Bucharest.
ELI-NP is part of the pan-European Extreme Light Infrastructure (ELI) project.1 The European Strategy Forum on Research Infrastructures (ESFRI) has recognised the importance of the emerging high-power ultra-short duration pulsed laser-based research for developing cost- and size-effective technologies for basic science research and for applications of societal relevance, and it included the ELI project on its Roadmap in 2006. In 2016, the ELI project was considered to have reached enough maturity to become an ESFRI Landmark. To stimulate a faster development of the field, the ELI project aims at developing a research infrastructure that should ensure the leap from laboratory-based to facility-based research. The ELI research infrastructure is a novel concept, being distributed over three pillars in different countries with complementary state-of-the-art equipment and research objectives: ELI-Beam lines in Czech Republic, ELI-ALPS in Hungary and ELI-NP in Romania2.
Two well-established scientific communities – laser physics and nuclear physics – have joined their efforts at ELI-NP to consolidate the field of research called Nuclear Photonics, based on the interaction of extreme electromagnetic radiation with matter. At the core of the ELI-NP research infrastructure, there are two main systems that will deliver beams with unprecedented parameters: a high-power laser system consisting of two arms of 10PW each, able to produce high irradiance (1023 W/cm2) laser pulses, and an intense gamma beam system delivering quasi-monochromatic beams with high spectral density of about 104 photons/s/eV.
ELI-NP is a multidisciplinary research infrastructure. The beams provided by the two systems allow for the performance of advanced research studies in a broad range of research fields, such as: nuclear physics, laser and plasma physics, optics, quantum electrodynamics, material science, biophysics, and life sciences.
Aside from its scientific goal to promote excellence in research, ELI-NP also aims at reducing the ‘brain drain’ phenomenon by implementing in Romania a large research infrastructure relevant at international level, with state-of-the-art instrumentation, strongly embedded in the European Research Area. It will act as an attraction pole for high-tech industries through capitalisation on the scientific and technological uniqueness of the ELI-NP infrastructure and on the scientific talents concentration that already exists on the Magurele Physics Platform.
Modern buildings for unique instruments
ELI-NP includes more than 33,000m2 of high-quality and energy-efficient buildings for the installation and operation of the experimental systems. The buildings have been operational since 2016. The Heating, Ventilation and Air Conditioning (HVAC) system, operating a total volume of air of about 270,000m3,is based on a geothermal system – the largest such system in Europe. The ground heat exchanger network contains 1080 boreholes 125m deep for a total length of 135km. With a total installed power of more than 6MW, the HVAC system was designed to run continuously for 365 days a year, 24 hours a day.
To ensure the very strict requirements of the experimental setups concerning protection against vibrations, the laser system, the gamma beam system and the experimental setups are installed on a single anti-vibration reinforced concrete platform supported by a network of over 1,000 springs and dampers.
The high-power lasers are installed in a ISO7 clean room with a surface of 2,800m2 (see Fig. 2) where the temperature is maintained to 0.5°C stability and the relative humidity is kept in the range of 30-55% while the whole area is over pressurised to prevent outside contamination.
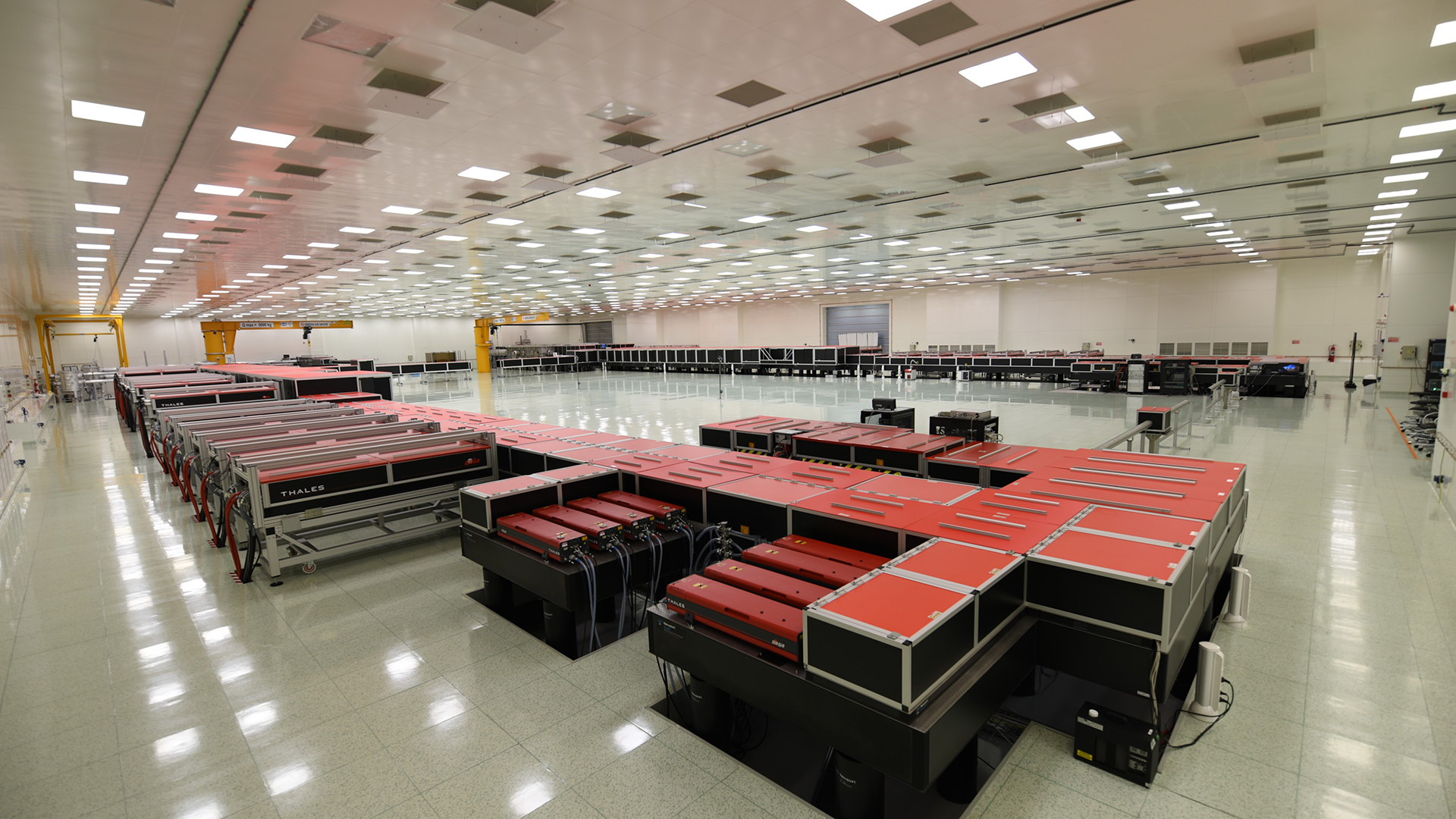
The most powerful lasers in the world
The High Power Laser System (HPLS) consists of two laser arms with a common front end. Each arm can deliver laser pulses with three different power levels and repetition rates: 0.1PW at 10Hz, 1PW at 1Hz and the maximum power level of 10PW at one pulse per minute. The laser system is of Ti:Sapphire type, generating infrared pulses of extreme light (central wavelength of 820nm) of ultra-short duration (25fs). The achievement of this unprecedented power level was permitted by the 1985 discovery of the Chirped Pulse Amplification technique, for which Gerard Mourou and Donna Strickland received the Nobel Prize in Physics in 2018. The uniqueness of the HPLS system is due, in first part, to the generation of 10PW laser pulses, but also to the availability of two such beams that can be used in separate experimental setups or in the same setup, allowing for premiere fundamental physics studies and novel applications.
HPLS was designed and built by Thales Optroniques France and Thales Systems Romania. In developing the system, several technical bottlenecks in the production of world premiere critical components were successfully solved, such as: the development of the 100J energy pump lasers, the production of large diameter (20cm) Ti:Sapphire crystals, and metre-size gratings for the laser compressors.
The 10PW laser pulses at the exit of the compressors are shaped as highly uniform intensity disks with 550mm diameter, to avoid the damage of the optical components in the compressors and transport lines that follow, and less than 10 microns in thickness due to the short duration (25fs) of the pulse. To achieve large irradiance values, the quality of the laser pulses has to be preserved throughout their transport from the laser to the interaction chambers where the experimental setups are installed. For this purpose, a dedicated Laser Beam Transport System (LBTS) for 10PW laser pulses was developed – a world-first achievement. Laser pulses are travelling in vacuum (10-6 mbar) inside 0.8m diameter aluminium pipes and their direction is stirred towards the interaction chambers with flat mirrors of about 1m diameter. One section of this transport system offers unique capabilities by hosting a 10PW 30m long focal length mirror for acceleration of high-energy, good-quality electron pulses in gaseous targets (see Fig. 3).
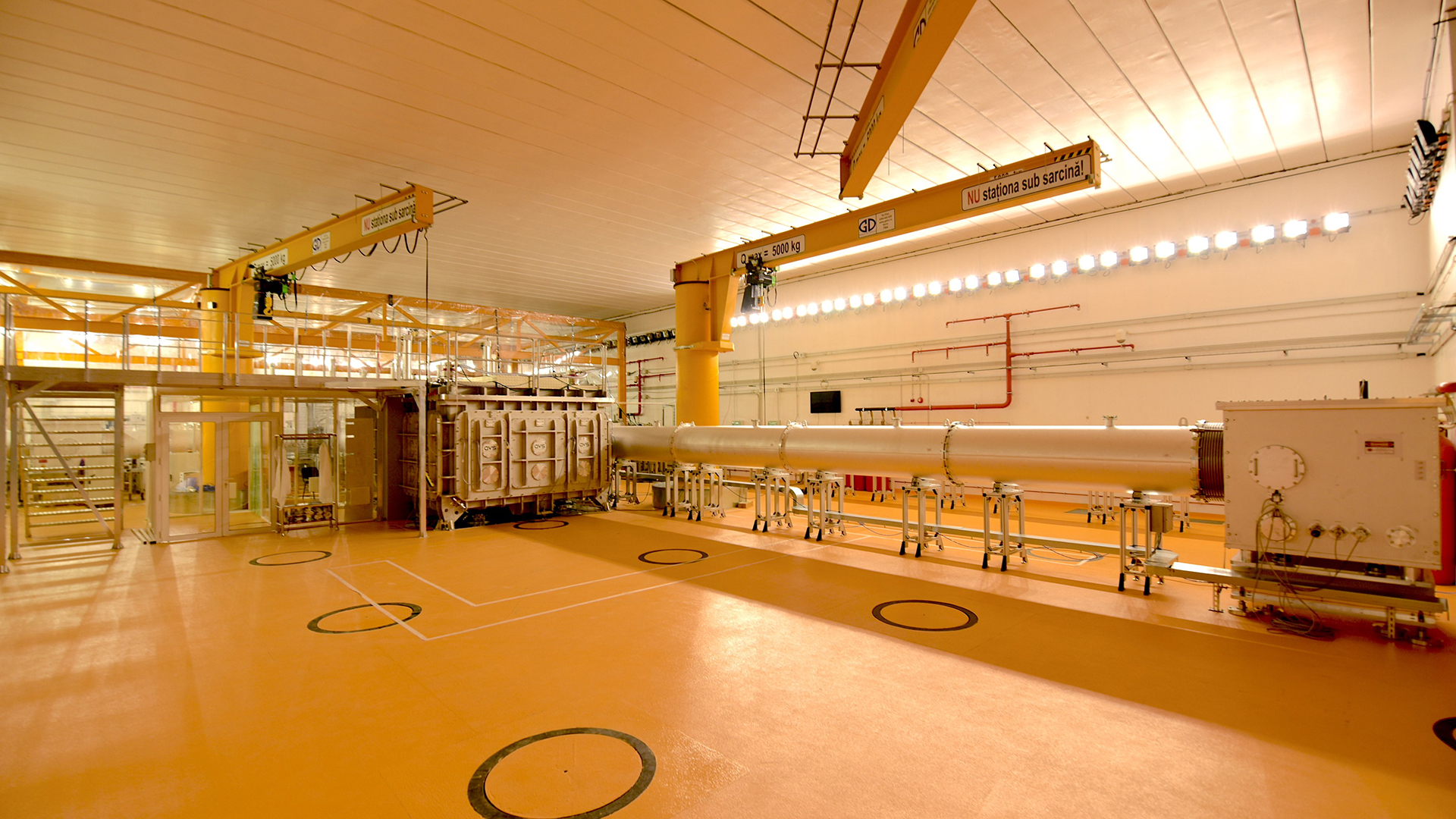
The commissioning of all the outputs, 2 x 0.1PW, 2 x 1PW and 2 x 10PW, of HPLS was successfully performed in 2019. The successful demonstration of the operation of the combined HPLS and LBTS at 10PW power level was performed publicly in 2020 during the inaugural 10PW Laser and Users Symposium: Moving into Unchartered Territories.
A very intense quasi-monochromatic gamma-ray beam
Intense gamma-ray beams will be produced by the Variable Energy Gamma (VEGA) System. The basic concept on which the system operates is the Inverse Compton Scattering (ICS) of low-energy photons (visible or infrared) on highly relativistic electron beams. In ICS, the photons are gaining energy rather than the electrons as happens in the standard Compton scattering. This is the most efficient energy transfer process in nature and photons can gain millions of times in energy, visible or infrared photons being transformed in gamma rays.
The VEGA System is a high-luminosity photon-electron collider under construction by Lyncean Technologies Inc. from USA. Electron bunches are produced by a warm linear accelerator with energies in the range 234-742 MeV. These electron bunches are then injected in a storage ring where they are circulated at high frequency. Low-energy photons produced by a laser are injected in a high-finesse optical cavity where laser power is built-up resonantly at a frequency matching the one of the storage rings. Photons and electrons collide in the storage ring. Photons will be generated with two wavelengths: ~1μm (infrared) and ~0.5μm (green). Following an almost head-on collision of the photons and electrons, the resulting gamma-ray beams emitted on the electrons direction of propagation will cover two energy ranges, 1-10MeV and 2-19.5MeV, when using the infrared or the green light, respectively.
The VEGA System will define the field of gamma beam systems by delivering intense quasi-monochromatic gamma-ray beams with relative bandwidth of less than 0.5%, spectral density of more than 5000 photons/s/eV, high degree of linear polarisation of more than 95% and small transverse dimension in the millimeters range.
Experiments with extreme light
The nature of the two extreme light sources at ELI-NP is of different type. In the case of HPLS, the unprecedented power level of 10PW leads to extreme irradiances (1023 W/cm2) of light when focused on a small area, resulting in an extreme light-matter interaction that breaks down matter into its most intimate constituents to nuclear level. Gamma-ray beams, on the other hand, are extreme by the energy of gamma-ray photons, that is millions of times higher than that of the photons from HPLS. This feature allows gamma-ray photons to interact with nuclear matter and initiate photonuclear reactions to study the properties of atomic nuclei.
The availability of such extreme beams of electromagnetic radiation opens new opportunities for advanced research topics on both fundamental and applied physics. One of the first tasks of the ELI-NP researchers was to identify the research topics to be addressed in the first years of running the systems and to design the corresponding experimental setups. Given the novelty and the uniqueness of the beams available at ELI-NP, a broad collaboration of more than 100 experts from universities and research institutes around the world was involved in this work. The results were published as a special issue of Romanian Reports in Physics Vol. 68 (2016).
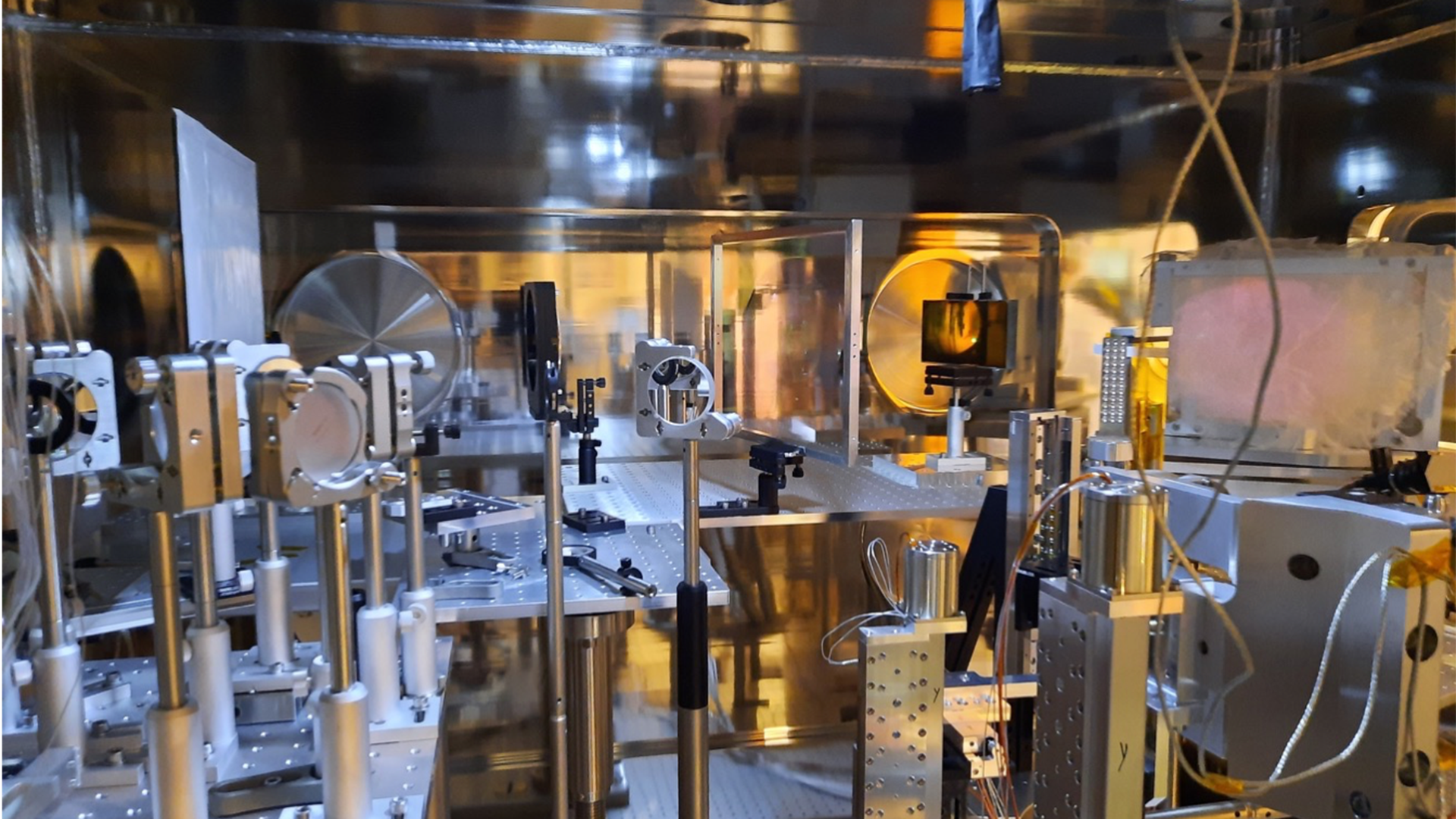
High power lasers provide new routes to production of high-intensity short-duration secondary radiation pulses and thus open new perspectives for fundamental and applied physics research. The first experiments started at ELI-NP in 2020 at the 100TW laser power level. In 2021, commissioning experiments were performed at both 100TW and 1PW (see Fig. 4) power levels, demonstrating a high reliability of the system and good quality of the laser beams. Parallel operation of the systems’ two arms allowed for almost double the available beam time in 2021. Commissioning experiments with high-power lasers aim at measuring the magnitude and scaling of the achievable laser irradiance via particle acceleration and laser-γ conversion efficiency. A better understanding and control of high-intensity short-duration accelerated laser-driven ion beams will be performed with solid and gaseous targets at different laser power levels. The possibility of using two high-power laser beams will allow for the performance of astrophysical interest nuclear reactions in hot and dense laser plasmas similar to stellar environments.
The outstanding characteristics of the gamma beams at ELI-NP will allow for sensitive Nuclear Resonance Fluorescence experiments to determine directly and model independently key information about the nuclear excited states, such as: excitation energy, spin quantum numbers, parities, branching ratios, level widths and gamma decay branching ratios. Electric dipole response of nuclei will be studied consistently below and above the particle emission thresholds (see Fig. 5). The study of rare nuclei available in nature in extremely low abundancies, such as p–nuclei, or of highly radioactive samples of actinides will become possible at ELI-NP. Measurements of reaction cross sections of astrophysical interest will be performed via γ,p or γ,α photo–disintegration processes.
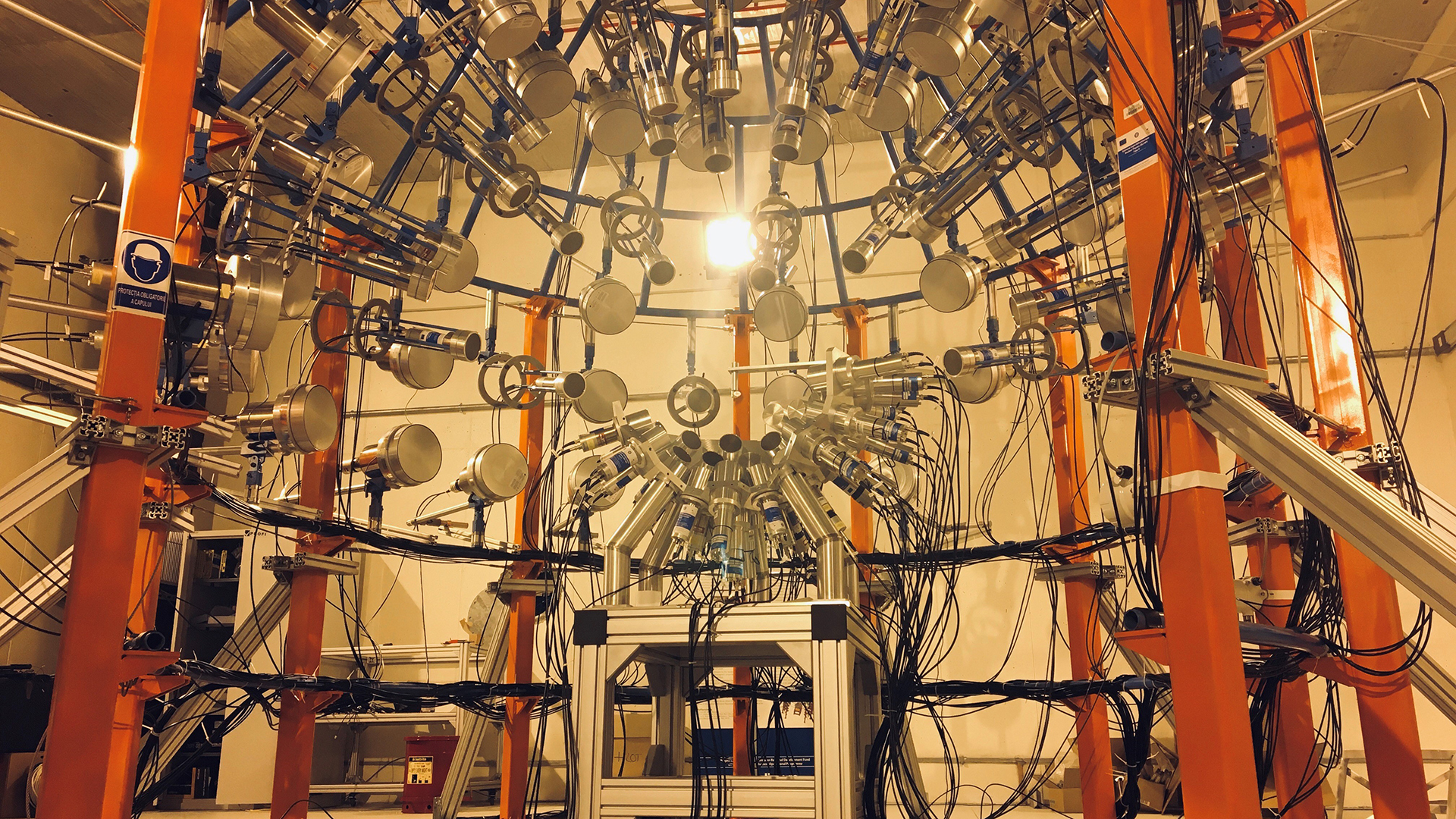
A broad range of applied research topics is also considered. The biomedical research programme will investigate new possibilities concerning laser-driven production of radiotherapy relevant particle beams; radiobiological effects of ultra-short secondary beams useful for the emerging flash radiotherapy; medical imaging with laser-driven X-ray sources; and medical radioisotope production. The behaviour of materials in high-intensity radiation fields simulating the extra-terrestrial environment are of interest for the space industry. Highly penetrant gamma rays will allow for the study of applications in 3D CT elemental scans relevant for industrial use and Sensitive Nuclear Materials identification.
Summary
ELI-NP is currently running commissioning experiments with high power lasers. The 100TW and 1PW experimental setups are already in an advanced stage of commissioning. The commissioning of 10PW experimental setups will start in 2022 and the experiments with gamma-ray beams will be performed in 2023, after the acceptance of the VEGA System. Starting with 2022, the facility will enter gradually, as the commissioning experiments are completed, a user facility regime, in which user experiment proposals will be evaluated and accepted based solely on scientific merit.
Acknowledgements
The ELI-NP project Phase II is co-funded by the European Union through the European Regional Development Fund and by the Romanian Government through the Competitiveness Operational Programme.
References
1 G.A. Mourou, G. Korn, W. Sandner, J.L. Collier (eds.) ELI – Extreme Light Infrastructure: Science and Technology with Ultra-Intense Lasers Whitebook, (THOSS Media GmbH, 2011)
2 ELI-NP White Book, www.eli-np.ro/whitebook.php
Please note, this article will also appear in the ninth edition of our quarterly publication.