Advocating for the importance of healthy soil, Vineland Research and Innovation Centre explains its role in the future of food security, conservation and agricultural sustainability.
Vineland Research and Innovation Centre is a uniquely Canadian results-oriented organisation dedicated to horticulture science and innovation. Delivering innovative products, solutions, and services, the Centre provides an integrated and collaborative cross-country network that advances Canada’s research and commercialisation agenda.
As part of this dedicated effort, Vineland advocates for improving and maintaining soil health, sustainability and food security. Crucial to global agricultural productivity, water conservation, and a sustainable food supply, our soil must be protected. Experts from the Centre elaborate on the how and why.
How do soil carbon levels impact soil health and agricultural productivity, and how does this affect sustainability?
Soil health is defined as the ongoing ability of soil to function as a vital ecosystem for plants, animals, and humans. It is a living, breathing system determined by physical (e.g. texture, bulk density), chemical (e.g. available nutrients) and biological factors (e.g. organic matter, soil respiration). Soil carbon, a vital aspect of the biological property, is crucial in soil health, productivity and sustainability.
Carbon naturally exists in all soils, but levels vary based on factors such as production practices, soil disturbance, and texture. Enhancing microbial life using organic matter input and improved production practices helps build soil carbon, an essential component for agricultural productivity and farm sustainability. Soil carbon:
- Serves as a food source for micro-organisms, promoting mineralisation and returning nutrients to the soil for plant use and growth;
- Provides soil structure, aiding in the formation of aggregates that improve water-holding capacity, infiltration, and erosion resistance; and
- It acts as a storage system for nutrients and water, regulating their release to plant roots, preventing leaching, loss, and erosion, thereby protecting aquatic habitats, which can become contaminated with excess nutrients and sediment from soil erosion.
Prioritising the development of soil carbon enhances sustainable farming by reducing fertiliser and irrigation needs, fostering plant growth and sequestering atmospheric carbon dioxide.
How does the use of absorbent soil contribute to water conservation and soil erosion prevention?
Water conservation and soil erosion are interconnected. To better understand their relationship, let’s revisit the essence of soil. Soil is comprised of four key components: Minerals (e.g. sand, silt, clay), organic material, water, and air. When we look at healthy soil, 50% of the soil should consist of water and air, often referred to as ‘open space’, ‘pore space’ or, more specifically, ‘soil porosity’. One cubic metre of healthy soil can store up to 0.5m³ of water (or 500L).
Healthy soil can act like a sponge, absorbing and storing rainwater for prolonged periods. This stored water then becomes accessible to plants. This is critical for plant growth, especially for the newly planted, establishing vegetation facing water limitations, which can determine their success or failure.
Compacted soil prevents water from entering pore spaces, leading rainwater to become surface runoff or stormwater runoff. As it travels downhill, it carries loose soil particles, causing soil erosion and soil loss. Massive erosion results in muddy streams flowing into rivers. To minimise soil erosion, a focus on upstream soils is crucial. Enhancing porosity by reducing soil compaction enables the absorption of rainwater into the soil, decreasing surface runoff and the risk of erosion.
Improving soil health, including absorbency, in urban environments can save on costs for watering and tree replacement programmes, while contributing toward the establishment of healthy and resilient urban trees that can grow to canopy height and contribute vital ecosystem services, including heat mitigation, urban cooling, air filtration, and shading.
What are the key characteristics of soil that promote healthy tree growth, and how do you study this?
Good soil health results from the proper balance of physical, chemical, and biological properties that mutually regulate one another, forming a sustainable foundation for plant growth. Degradation of one soil parameter adversely affects the others, as evident in soils with low organic matter. Soil organic matter, comprising living organisms and organic residues, enhances soil porosity and reduces bulk density, facilitating increased water and air entry. This environment supports micro-organisms that break down organic matter and release nutrients, like nitrogen, for plant uptake. The absence of organic matter leads to negative impacts, such as nutrient deficiency, heightened compaction and reduced soil microbial activity.
Trees are large, long lived organisms that require greater soil volume, investment during the three to five-year establishment phase, and long-term maintenance as compared to other ornamental and landscape plants. Where soil is heavily compacted, low in organic matter, or has typical urban issues like high salt content resulting from the use of de-icing salts, trees often cannot penetrate and establish adequate root systems to support effective growth, limiting their ability to become large, healthy trees. Poor soil health often leads to the gradual decline and eventual removal of newly planted trees in the urban environment, typically occurring over five to ten years as the tree exhausts the limited resources available in unmaintained urban soil and eventually dies.
The need to understand tree establishment and its interaction with soil health was the impetus to develop the TreeCulture Research Park. This state-of-the-art facility was designed to allow researchers to study trees and soil under semi-controlled conditions. Most research involving trees occurs either in the field, where conditions can be pretty unpredictable, or in the lab, where researchers work to replicate real-world conditions by building and testing model systems in benchtop experiments. The TreeCulture Research Park offers the best of both, allowing scientists to conduct research trials and experiments at scale while controlling inputs and testing specific soil treatments under natural environmental conditions.
Each tree is planted into a specially designed tree compartment buried below ground and filled with particular soil mixtures that are being trialled and tested before use in real-world applications. Compartments measure 4.5×4.5m wide and 1m deep, giving tree roots plenty of space to extend and grow. All compartments are outfitted with soil sensors, measuring everything from soil temperature, moisture and pH to oxygen content and water availability. Sensors collect soil data continuously and transfer information to the cloud, which our research team monitors and analyses. The inaugural research experiment assesses the effectiveness of low-impact developments to optimise stormwater infiltration and storage and maximise tree establishment and growth.
What are the key considerations in breeding crops for adaptation to Canadian climates and growing conditions?
Climate change has presented several additional challenges for growers. Whether it is the extreme heat, drought, unexpected frost events, or new and emerging pests and diseases, growers are looking to newer, adapted varieties to help withstand these pressures. Here at Vineland, we take a unique approach to breeding that combines the considerations from the entire value chain and cutting-edge scientific expertise & tools. These tools include:
Development of a consumer preference map:
A statistical model that links discrete sensory attributes & their contribution to consumer preference or product optimisation. This tool is used in the selection process to identify material predicted to have high consumer acceptance;
Biochemical selection tools:
Using a process of biochemical selection, we can incorporate traits like the aroma volatile profiles of consumer-preferred varieties into modern cucumber genetics; and
Patented non-GMO technology enabled trait development:
Deep Variant Scanning (DVS) technology quickly turns off genes in practically any seed-crop species. This can create new traits such as stress tolerance and disease resistance. Based on mutagenesis, this technique is recognised globally as a non-GMO technology.
In turn, an adapted crop should have the ability to respond to these pressures to minimise losses. In the case of fresh food, an adapted variety should have the appearance, taste, aroma, and texture preferred by consumers. Furthermore, the adapted variety must perform well throughout harvesting, packaging, and shipping to enable it to perform in the retail environment and maintain its freshness and visual appeal. For processed crops, a new variety should meet the parameters of the food processors (e.g. size, shape, nutritional content) so that minimal modifications to their equipment are required. With the growing demands of food production, developing adapted varieties is one consideration to tackling food security, while an alternative approach is reducing waste.
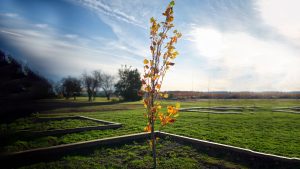
What is critical when determining the waste streams for various agricultural products and considering how to repurpose said waste into more productive processes?
Several factors determine the viability of repurposing fruit and vegetable by-products (e.g. skins, peels, stalks, grade-outs) into value-added products. These considerations include:
- Volume of by-products available;
- Availability and seasonality of the by-products (year-round or specific time period);
- Perishability of the by-product;
- Purity of the by-product stream (mixed or single ingredient);
- Quality of the by-product stream (food grade or non-food applications);
- Minimal transportation from the grower facility to the processing facility;
- Pre-processing requirements (water, debris, seed removal, etc.);
- Potential functionality of upcycled by-product (fibre, enzyme activity, nutrients); and
- Potential applications and implementation into value-added products (e.g. food ingredients, fruit and vegetable powders, coatings, soil amendments, nutrient supplements).
Each by-product stream needs to be evaluated individually for its optimal upcycling use. Vineland recently assessed underutilised by-products from the top seven Canadian horticultural crops — carrots, apples, potatoes, field tomatoes, greenhouse tomatoes, cucumbers, and onions. Our findings identified crops with limited processing markets ideal for by-product streams, including unavoidable waste from produce processing.
However, linking producers with companies who can utilise these by-products remains a challenge. This is where Vineland’s R&D support for product development, market analysis, and supply chain connections can help fill an important gap.
How can the life cycle assessment (LCA) results inform decision-making related to sustainable food systems?
The entire food system operates through various stages and involves multiple players in a complex network. Each stage contributes to environmental footprints, including greenhouse gas emissions, energy, and water usage. The global food supply chain is responsible for 13.7 billion tonnes of CO2 (26% of total anthropogenic emissions), utilising 50% of habitable land, 70% of global freshwater, and 78% of global eutrophication (Ritchie, n.d.).
Amidst the challenge of increasing food demand due to population growth and climate change impacting agriculture, there’s a pressing need to reduce the food system’s environmental impact. Recognising this environmental burden is a crucial initial step toward achieving a sustainable food system.
Life cycle assessment is a science-based method to map and gauge the environmental impact of food across the agri-food system, effectively painting a complete picture of the ecological burden. More specifically, this analysis can articulate sustainable food systems in several ways, including:
- Mapping the food journey through various life cycle models, such as cradle-to-grave or cradle-to-farm gate, based on specific requirements;
- Systematically measuring the environmental burden associated with the food system, including carbon footprint, greenhouse gas emission intensity, acidification, depletion of abiotic resources, ecotoxicity (freshwater, marine, terrestrial), eutrophication, human toxicity, and ozone layer depletion;
- Identifying the life cycle stage with the highest environmental footprint, offering insights for decision-making on food imports, domestic production, and emission reduction strategies;
- Pinpointing environmental footprint hotspots aids in developing effective mitigation strategies, using the initial LCA as a baseline for future studies; and
- Farmers adopting measures to minimise environmental footprints aligns with climate action and emission reduction goals set by governments, making them competitive in the downstream value chain, including distributors and retailers. This approach is relevant to the food processing industry and agribusiness.
Important linkages for sustainable food production: Soil health and food security
The poor establishment, growth and survivorship of trees in our landscapes, or underperforming crops in agricultural fields are indicative of soil health declines. The challenges affecting our urban forest parallel those of the agricultural and horticultural production industries, where an overuse of soil resources and general lack of investment in soil health has contributed toward agricultural soils that are highly compacted and lacking organic matter with poor infiltration and insufficient water and nutrients to support plant growth and productivity.
Compounded by the effects of climate change, these agricultural soils are subject to the same fate as our urban landscapes, where a lack of basic resources will limit root development, plant growth, productivity, and survival to the point at which vegetation, in this case the crops that make up our food, may fail to meet our widespread production and consumption needs. Where trees are large and long-lived, they have the unique capacity to demonstrate the impact of soil health over time in a way that short lived annual crops do not. What we see in our trees is demonstrative of the impacts we are likely to see in our food over successive crop cycles, with gradual declines in productivity and yield occurring year to year, rather than within a single tree.
Accordingly, Vineland’s history of soil health research, is increasingly relevant to our food and crop security- where building soil’s physical, chemical and biological health at a landscape level will contribute toward more sustainable, resilient and interconnected systems that support survival, establishment and growth of plants.
Please note, this article will also appear in the seventeenth edition of our quarterly publication.