Professor Federico Sanchez from the University of Geneva’s Department of Nuclear and Particle Physics looks at the history of neutrino experiments in Japan and how their successes are feeding the future.
Neutrinos have been a fascinating field of research, providing critical discoveries. In 1931, Wolfgang Pauli proposed an elusive particle, the neutrino, with almost no mass to explain some radioactive decay observations. Since then, neutrinos have contributed to the establishment of the Standard Model of Particle Physics.
The history of neutrinos is full of extraordinary discoveries, from the violation of the parity (physics laws are not the same for a mirrored Universe) to the determination of the three-particle families and the discovery of the neutral mediators of the weak interactions. The most recent discovery, neutrino oscillations, evolved rapidly from a plausible hypothesis during the second half of the 20th century to a well-established research topic.
As for many other particles in the Standard Model, there are three types of neutrinos. Neutrino oscillations are a quantum mechanics interference phenomenon produced by the fact that the three neutrino flavour states (the way the neutrino interacts) are not the same as the three mass states (the way the neutrino propagates in space). The difference in neutrino masses induces that the quantum phase varies differently during the travel of neutrinos from production to detection. These variations alter the proportion between the different neutrino species. Oscillations are a quantum mechanics effect observed at terrestrial distances contrary to typical laboratory-scale experiments. Neutrino oscillations are described in current models by a three-dimensional rotation governed by three rotation angles, the two mass differences between the three neutrino masses and a parameter differentiating neutrinos from its antiparticles, the anti-neutrinos.
The Kamiokande experiment
Research in Japan has been fundamental in the determination of the neutrino oscillation phenomenon. This research has been made possible mainly by a scientific programme that conjugates dedication, tradition, and some degree of good luck. The programme started in 1983 after one year of construction of the Kamiokande experiment.1 The experiment’s name stands for Kamioka, a village in the mountains close to Toyama, and the suffix ‘neutron decay experiment’ (NDE). The experiments were operated under the responsibility of the Kamioka observatory1 depending on the Institute of Cosmic Ray Research of the University of Tokyo. The Kamioka observatory is a neutrino and gravitational wave observatory located in the Mozumi Mine of the Kamioka Mining and Smelting Co. The mining operations ceased in 1985 and the underground facilities were refurbished to accommodate experimental setups.
The Kamiokande experiment consisted of a large cylinder, approximatively 16m in height and diameter. The purpose of the experiment was to look for the radioactive disintegration of the proton. Protons are described in the current Standard Model as stable particles. Once produced and in absence of interactions, a proton will remain a proton. The observation of proton disintegration will thus induce a revolution on our current understanding of Nature.
The Kamiokande experiment did not find any proton decay. However, during its operation it was able to detect neutrinos from the explosion of a supernova in 1987.2 Supernovae are stars at the end of their life cycle. When all the nuclear fuel is consumed, the supernovae die with a big explosion that emits a large number of neutrinos. This discovery was the subject to the Nobel Prize awarded to Professor Koshiba in 2002.
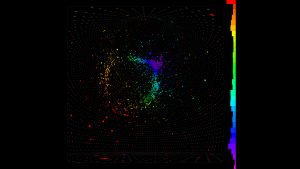
Solar neutrinos
Kamiokande also looked for neutrinos produced in the nuclear fusion reactions inside the Sun, proving that they were actually produced by the Sun looking at their direction in 1988.3 Solar neutrinos have been subject to controversy after the first neutrino detection by the Homestake experiment in the late 1960s. The number of neutrinos detected was of the order of a factor of two smaller than the number expected by the model of solar dynamic initiating the so-called ‘solar neutrino problem’.
Kamiokande proved to be an excellent multipurpose experimental device that planted the basis for future developments. The keys to the success were its large mass that enhances the probability of neutrinos to interact with the detector and the sample of possible protons to decay and a simple detection method based on the light emitted by particles moving close to the speed of light in the water. Large photosensors attached to the tank walls detected the emitted light. This configuration allowed for very cost-effective instrumentation of large detector volumes. The last ingredient to its success was the fact that the detector was located in an underground mine to shield it from unwanted muons produced by cosmic ray interactions with the earth atmosphere.
Super-Kamiokande
The next experiment, Super-Kamiokande,4 was approved in 1991 following the success of Kamiokande. The new detector followed the same design and operation principles but with 20 times more mass. The number of neutrino interactions increased accordingly but so did the total number of potential proton decays. The principal physics goal shifted from proton decay into solar and atmospheric neutrino detection, enhancing the multipurpose capabilities. At that time, neutrino oscillation was the most plausible explanation to the ‘solar neutrino problem’, a hypothesis which required experimental confirmation.
The experiment started taking data in 1996, just five years after its official approval, and – as had happened with Kamiokande – the surprise came from other neutrinos; in this instance, those produced by cosmic rays. After two years of operation, the experiment published compelling evidence of neutrino oscillations using the so-called ‘atmospheric neutrinos’.5
Professor Takaaki Kajita was awarded the Nobel Prize for physics for this discovery in 2015. Kajita shared the Prize with Professor Arthur B McDonald, who had demonstrated neutrino oscillation with neutrinos from the sun in the SNO experiment. SNO is an impressive apparatus, comprising a cylinder 40m diameter and with 40m depth containing 50,000 tons of ultra-pure water read by a total of 11,200 photosensors 20 inches in diameter.
Precision and diversification
The Super-Kamiokande detector is still in operation 24 years later and it continues to provide world-class physics results thanks to the pragmatic approach. Much of its longevity comes from the fact that there is no other experiment of this scale and precision and, moreover, because the Japanese neutrino programme has diversified around it. After the atmospheric neutrino oscillation discovery, interest shifted to the precise determination of the leading parameters controlling the oscillations. Precision measurements need control of all the aspects of the experiment. This task is not simple; neutrinos produced by cosmic rays suffer uncertainties in the modelling of the cosmic-rays energy and its particle composition. The idea to overcome these difficulties was to study the neutrino oscillation phenomena using control sources of neutrinos. Neutrinos can be produced in accelerators in physics processes similar to cosmic rays but under controlled conditions. While Super-Kamiokande continued taking data, a new project emerged to send neutrinos from the particle physics laboratory KEK in Japan. The idea was to reuse a low energy proton beam to produce neutrinos 250km away in Tsukuba (Japan). The new project, ‘KEK to Kamioka’ (K2K),6 used the technology to produce the neutrinos known since the 1960s and it demonstrated that neutrino detection is possible at very long distances.
The K2K experiment ran from 1999 until 2004 and set the foundations of long-baseline experiment technology: the time synchronisation between production and detection based on GPS technology, and the importance of neutrino detectors close to the production point in order to control neutrino production. The K2K experiment ran parasitically to Super-Kamiokande that was minimally modified to adapt to the new neutrino sources. This modification added a new line of research that became the dominant one over the following years. K2K was the first experiment to demonstrate neutrino oscillations with artificial neutrino sources.8
JPARC
From the very beginning, the limitations of the K2K experiment were clear to the community. The accelerator was not able to produce a large number of neutrinos, thus reducing the precision of the measurements. This limitation was the origin of the T2K (Tokai to Kamioka)9 experiment, born synergistically to a new accelerator facility in Japan, the Japan Proton Accelerator Research Center (JPARC).10 JPARC provided a high-intensity proton beam with higher particle energy. The facility was located a mere 50km from the old accelerator. The concept of the experiment was similar to that of K2K, with 50 times higher event statistics.11
The goal was to measure one of the angles associated with the oscillations. This angle, measured to be small, was difficult to reach experimentally. To achieve this goal, T2K needed to detect the transition between two types of neutrinos (muon to electron). Until this moment, the experiments relied on the disappearance phenomena (i.e. detecting fewer neutrinos of one species than expected). The construction of the new beam started in 2004. The first beam was delivered in 2009. It took two years of beam operation to detect the above-mentioned transition, determining a non-vanishing value of the third oscillation angle.12
These results, and the ones from K2K and SuperKamiokande, were awarded the Breakthrough Prize for fundamental physics which is awarded to those experiments that have made significant contributions to the development of neutrino oscillations.
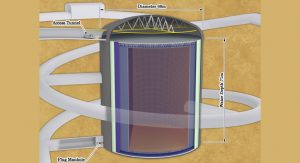
CP violation
T2K is still running and producing excellent results. In 2020, the T2K collaboration presented the first indication that neutrinos and their antiparticles might oscillate differently.13 If this measurement is confirmed, it will be the first evidence of the difference between neutrinos and their antiparticles, the anti-neutrinos – the so-called ‘CP violation’.
This particle-antiparticle differential phenomenon has been known for quarks (the inner components of protons and neutrons) since the 1960s. The discovery of the parallel phenomenon in neutrinos is a critical ingredient to understand the matter-dominance in our Universe, or why our Universe is made of matter and not antimatter. The confirmation will again come from larger samples of data. The final determination of the CP violation requires a more powerful beam as well as a larger neutrino detector. Following the Japanese neutrino tradition, this process is done by upgrading one of the elements in the experiment. After of years of beam development, it is now the time to upgrade the Kamiokande saga.
Hyper-Kamiokande
The new experiment, called Hyper-Kamiokande, has been recently approved by the Japanese government14 and it will start operation in 2026. The new detector is also a cylinder; it will be 70m in diameter and depth, containing 260,000 tons of water readout by 40,000 photosensors. This new detector will provide a factor of ten more neutrino interactions at a constant beam power. These unprecedented statistics will allow us to determine if neutrinos behave as anti-neutrinos. That means the experiment will collect more than the total accumulated number of neutrinos by the T2K collaboration in a single year.
The concept of the experiment has remained unchanged since the 1980s, although there are technological improvements that will enhance the experiment’s physics reach. One of these is the photosensors optimisation. In the meantime, T2K will continue to improve recent results and prepare for the next generation.
This adiabatic evolution has proven to be very fruitful. It allows researchers to concentrate on physics and less on technology developments. Over the last few decades, the Kamiokande-saga community has accumulated knowledge that makes the operation and analysis of the data more efficient. One of the relevant aspects to oscillation experiments is related to the neutrinos’ interaction with water. The neutrino-nucleus interactions require proper modelling of the nuclei. The needed level of precision has no precedent in nuclear physics. Both the K2K and T2K experiments have been a driving force in developing the models over the last two decades, with a close connection to the nuclear theory community.
The past and the future
All of this accumulated knowledge, reflected in numerical models, can be directly applied to the new detectors in order to improve the experimental results beyond the increased number of the detected neutrinos. Different aspects of the experiment, such as the modelling of the neutrino flux or the analysis of the light in the Kamiokande detector, migrated from one generation to the next, constituting the basis for the future experiment. The tradition is complemented by innovation, achieved by an active young generation of researchers who are implementing new developments such as the application of machine learning technology to the reconstruction tasks.
This approach’s success is demonstrated by the outstanding list of results generated during the last 25 years. Each of the stages of the Japanese programme provided excellent physics results including two Nobel Prizes and one Breakthrough Prize. The staging has worked in such a way that there has been almost no time when physics results have not been continuously flowing.
The approach has also permitted the experiment to lead some of the techniques required by these experiments: neutrino-nucleus interaction, neutrino beam modelling, and statistical models for the data analysis. This leadership has been made possible by the dedicated contribution of international partners from North America and Europe. The contributions from non-Japanese institutions goes back to the time of Kamiokande evolving into a truly international enterprise, with 14 nations involved in the design, construction, and scientific exploitation of the experiments. The portfolio of potential physics measurements has increased since the first Kamiokande experiment to proton decay and supernova neutrinos; Superkamiokande added solar and atmospheric and beam-neutrinos. HyperKamiokande will improve on all of them.
We should not forget that the history of the Japanese neutrino programme is already full of surprises, and in the future new and unexpected discoveries may change our understanding of the world of particle physics.
References
- http://www-sk.icrr.u-tokyo.ac.jp
- Hirata et al., (Kamiokande Collaboration), ‘Observation of a neutrino burst from the supernova SN1987A’. Physical Review Letters, 58 (14): 1490–1493
- Hirata et al., (Kamiokande Collaboration), ‘Real time, directional measurement of B-8 solar neutrinos in the Kamiokande-II detector’, Physical Review D 44 (1991) 2241
- Fukuda et al., (The Super-Kamiokande Collaboration), ‘The Super-Kamiokande detector’. Nuclear Instruments and Methods in Physics Research A, 501 (2–3): 418–462
- Fukuda et al., (The Super-Kamiokande Collaboration), ‘Evidence for oscillation of atmospheric neutrinos‘. Phys. Rev. Lett. 81 (1998) 1562-1567
- R. Ahmad et al., (SNO Collaboration), ‘Measurement of the Rate of νe + d → p + p + e− Interactions Produced by 8B Solar Neutrinos at the Sudbury Neutrino Observatory’. Phys. Rev. Lett. 87 (7): 071301
- https://neutrino.kek.jp/intro/k2k.html
- H. Ahn et al., (K2K Collaboration), ‘Measurement of Neutrino Oscillation by the K2K Experiment’. Phys. Rev. D 74, 072003 (2006)
- https://t2k-experiment.org
- http://j-parc.jp/c/en
- Abe et al., (T2K Collaboration), ‘The T2K Experiment’. Nucl. Instrum. Meth. A 659 (2011) 106-135
- Abe et al., (T2K Collaboration), ‘Indication of Electron Neutrino Appearance from an Accelerator-produced Off-axis Muon Neutrino Beam’. Phys. Rev. Lett. 107:041801, 2011
- Abe et al., (T2K Collaboration), ‘Constraint on the matter–antimatter symmetry-violating phase in neutrino oscillations’. Nature. 580: 339–344. 15 April 2020
- https://www.nature.com/articles/d41586-019-03874-w
Please note, this article will also appear in the fifth edition of our quarterly publication.