A research team from Linköping University has made headway in discovering superconductive materials that are useful in real-world situations.
How was this superconductive material discovered?
Scientists have discovered that magnesium diboride becomes superconductive at a higher temperature when it is stretched. This discovery is a big step in the scientific community, as it possesses potential toward finding superconductive materials that are useful in everyday situations.
“Magnesiumdiboride or MgB2 is an interesting material. It is a hard material that is used for instance in aircraft production and normally it becomes superconductive at a relatively high temperature, 39K, or -234°C,” explained Erik Johansson, who recently completed his Doctorate at the Division of Theoretical Physics.
“Magnesium boride has an uncomplicated structure which means that the calculations on the supercomputers here at the National Supercomputer Centre in Linköping can focus on complex phenomena like superconductivity.”
Erik Johansson is the principal author of an article published in the Journal of Applied Physics that has attracted significant attention, with the editor noting that the results are particularly important for future applications.
How can this material be useful in everyday situations?
Access to renewable energy is fundamental for a sustainable world, but even renewable energy disappears in the form of losses during transmission in the electrical networks. These losses are due to the fact that even materials that are good conductors have a certain resistance, resulting in losses in the form of heat.
For this reason, scientists worldwide are trying to find materials that are superconductive and conduct electricity with no losses at all. Such materials exist, but superconductivity mostly arises very close to absolute zero.
Years of research has resulted in complicated new materials with a maximum critical temperature of 200K, which is approximately -73 °C. At temperatures under the critical temperature, the materials become superconductive. Research has revealed that superconductivity can be achieved in certain metallic materials at extremely high pressure.
Thus, if scientists are successful in increasing the critical temperature, there will be greater opportunities to utilise the phenomenon of superconductivity in practical applications.
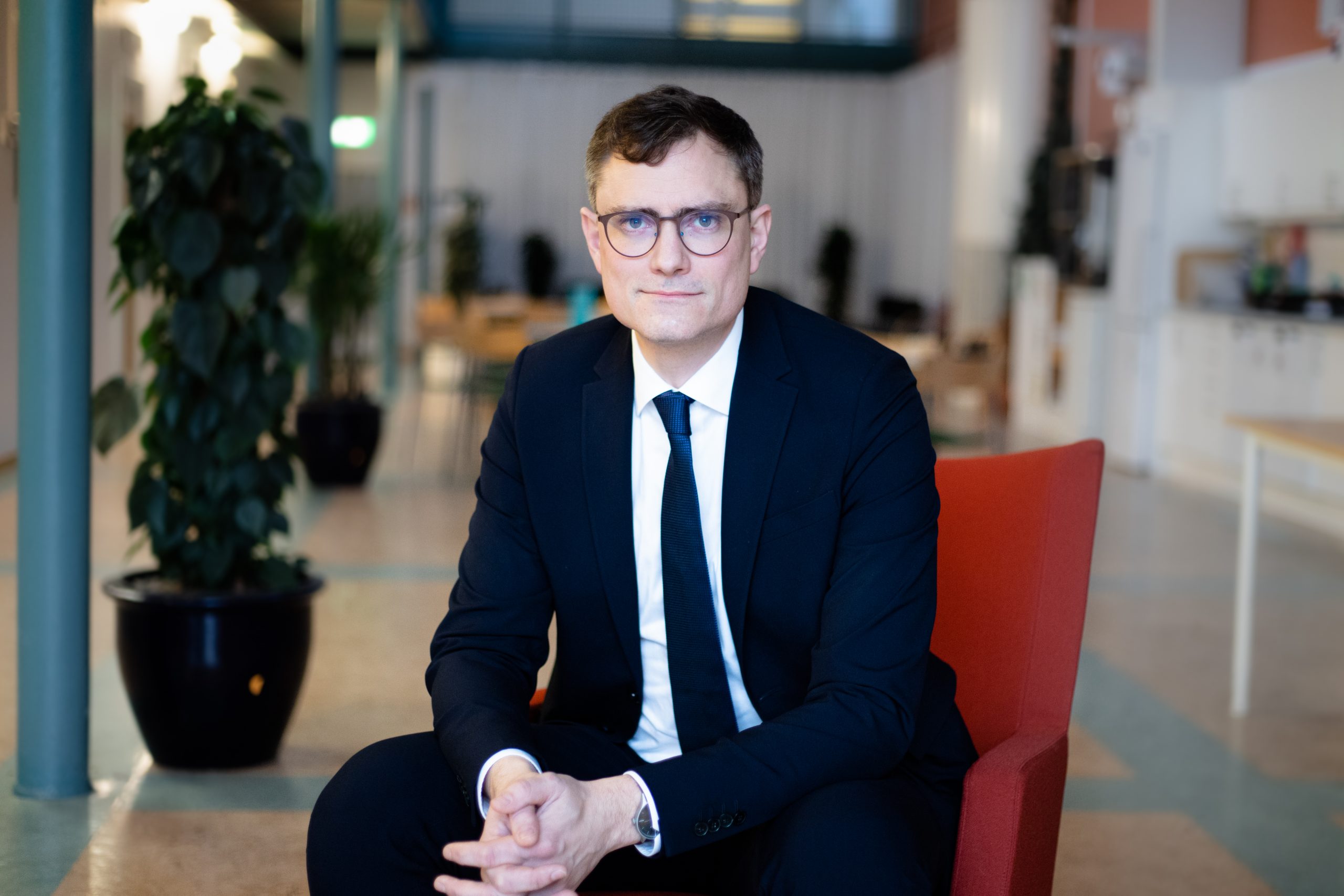
Photo credit: Charlotte Perhammar.
How to scientists plan to achieve this?
Johansson noted: “The main goal is to find a material that is superconductive at normal pressure and room temperature. The beauty of our study is that we present a smart way of increasing the critical temperature without having to use massively high pressure, and without using complicated structures or sensitive materials.
“Magnesium diboride behaves in the opposite way to many other materials, where high pressure increases the ability to superconduct. Instead, here we can stretch the material by a few percent and get a huge increase in the critical temperature.”
In the nanoscale, the atoms vibrate even in really hard and solid materials. In the scientists’ calculations of magnesium diboride, it emerges that when the material is stretched, and the atoms are pulled away from each other and the frequency of the vibration’s changes.
This means that in this superconductive material, the critical temperature increases – in one case from 39K to 77K. And if magnesium diboride is instead subjected to high pressure, its superconductivity decreases.
The discovery of this phenomenon paves the way for calculations and tests of other similar materials or material combinations that can increase the critical temperature further.
“One possibility could be to mix magnesium diboride with another metal diboride, creating a nanolabyrinth of stretched MgB2 with a high superconductive temperature,” concluded Björn Alling, Senior Lecturer at the Division of Theoretical Physics, and director of the National Supercomputer Centre at Linköping University.
The research has been funded by the Knut and Alice Wallenberg Foundation, the Swedish Research Council and the Swedish Foundation for Strategic Research, as well as others. It has been conducted with support from the government’s strategic venture, Advanced Functional Materials (AFM), at Linköping University.
To keep up to date with our content, subscribe for updates on our digital publication and newsletter.