For a society that thrives on renewable energy, it is essential that excess renewable energy is converted into chemical fuels.
Hydrogen peroxide (HP) is an important chemical that is produced yearly on a 2m ton scale and is relatively expensive for a simple molecule that consists of only four atoms. HP is used in in small quantities as an oxidiser and/or an antibacterial agent in many products such as disinfectants, stain removers, clog-removers, bleach, hair colour agents, toothpaste, facial and body creams, mouth wash, antibacterial soap, antiseptic rinse products, odour removers, and contact lens solutions. In addition to this, large scale applications include the textile and paper industry, and the paper recycling process requires high amounts of peroxide in order to oxidatively remove ink. HP is considered to be a green oxidant because all of its atoms are transferred to the substrate with water being its only side product. However, its synthesis is not the most sustainable process.
HP is exclusively produced by the chemical industry via the anthraquinone procedure.1-3 In this process, HP is produced in a stepwise batch process that requires the usage of stoichiometric amounts of anthraquinone, and a cumbersome separation of these reagents. The synthesis of HP therefore is energy intensive and generates a lot of waste as a result of many different and complex side reactions that take place during the anthraquinone process. Preferably, it would be a lot better if HP could be produced in an electrochemical manner using a catalytic and sustainable process. A proof-of-concept design for such an application was recently published by the group of Haotian Wang, who produced pure aqueous H2O2 solutions up to 20% by weight.4
It is important to mention that it is not easy to find a good catalyst for the electrochemical production of HP. Reduction of dioxygen to water is more beneficial from a thermodynamic point of view than the reduction of dioxygen to HP which is an high energy intermediate on route to water. A catalyst is needed that very easily catalyses the first sequence of steps to produce HP, while the second steps towards HP proceeds over a large barrier (see Fig. 1).
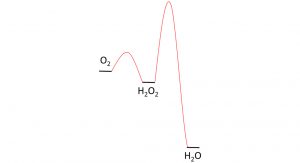
Under non-electrochemical conditions, HP does not react with itself to produce oxygen and water spontaneously. The elephants toothpaste experiment is a famous example of such a reaction (see Fig. 2). The spontaneous decomposition of HP in a reaction with itself is highly exothermic and catalysed by many transition metal catalysts and metal surfaces. These should be rigorously avoided as catalysts, or components, of the electrochemical cell.
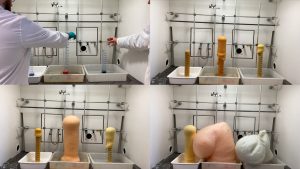
Oxidised carbon electrodes are good catalysts to produce HP selectively. This latter selectivity is important to get the most HP out of the electrochemical reactor without moving to huge currents and/or applied potentials. Mercury catalysts appear to do significantly better, but the sheer toxicity of this material will most likely hamper its commercial success.1-3
In the group of Hetterscheid, the electrochemical synthesis of HP is studied in the presence of homogeneous copper catalysts. It was found that especially Cu(tmpa) is a very fast catalyst and is capable of reducing almost two million molecules of dioxygen to peroxide within one second.5 Fig. 3 shows the structure of the catalyst and the proposed mechanism that heavily builds on upon lots of fundamental studies by the group of Kenneth Karlin who have studied the chemistry of Cu(tmpa) and many related compounds with oxygen in great detail.6
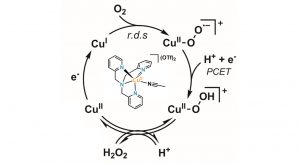
Fig. 3 Proposed mechanism of HP formation upon electrochemical reduction of oxygen in the presence of the Cu(tmpa) catalyst, (depicted in the middle of the catalytic cycle)
The selectivity towards HP produced by the electrochemical reduction of oxygen mediated by Cu(tmpa) lies far above 90% at a voltage that is close to the thermodynamic minimum. However, the efficiency quickly drops once the concentration of oxygen becomes depleted at the electrode surface, and the local concentration of HP becomes significantly larger. Under these conditions, the HP reduction rates become the dominant reaction, leading to very low selectivity. Fundamental studies wherein we aim to learn the design principles of these catalysts, which hopefully allow us to control, steer and adjust the activity of these catalytic systems are in progress. If successful, an approach such as this may allow for a more sustainable synthesis of HP for applications ranging from simple household products to large scale industrial applications.
For a society that thrives on renewable energy, it is essential that society is able to convert excess renewable energy to chemical fuels that can then be consumed in times of scarcity and for applications that require high-energy dense fuels. Therefore, the most straightforward approach is splitting water through electrolysis to produce oxygen and hydrogen, whereas storage of energy in batteries suffers from very low energy densities. It is interesting to note that the volumetric energy density of hydrogen at 100 bar pressure and HP in a 33% solution in water are very similar, while HP (in contrast to hydrogen) does not need to be stored in a heavy pressurised vessel. However, before we can obtain HP as a fuel in large quantities and at a sufficiently low price, a lot more scientific research is required.
References
1 Campos-Martin, J. M., Blanco-Brieva, G, and Fierro, J. L. G. 2006. Hydrogen peroxide synthesis: An outlook beyond the anthraquinone process. Angewandte Chemie, International Edition, 2006, 45, 6962 – 6984
2 Edwards, J. K., Freakley, S. J., and Lewis, R. J. et al. 2015. Advances in the direct synthesis of hydrogen peroxide from hydrogen and oxygen. Catalysis Today, 2015, 248, 3 – 9
3 Siahrostami, S., Verdaguer-Casadevall, A., and Karamad, M. 2013. Enabling direct H2O2 production trough rational electrocatalyst design. Nature Materials, 2013, 12, 1137
4 Xia, C., Xia, Y., and Zhu, P. 2019. Direct electrosynthesis of pure aqueous H2O2 solutions up to 20% by weight using a solid electrolyte. Science, 2019, 336, 226 – 231
5 Langerman, M., and Hetterscheid, D. G. H. 2019. Fast Oxygen Reduction Catalyzed by a Copper(II) Tris(2‐pyridylmethyl)amine Complex through a Stepwise Mechanism. Angewandte Chemie, International Edition, 2019, 58, 12974 – 12978
6 Lucas, H., Meyer, G. J., and Karlin, K. D. 2010. CO and O2 Binding to Pseudo-tetradentate Ligand−Copper(I) Complexes with a Variable N-Donor Moiety: Kinetic/Thermodynamic Investigation Reveals Ligand-Induced Changes in Reaction Mechanism. Journal of the American Chemical Society, 2010, 132, 12927 – 12940
Dennis Hetterscheid
Associate professor
Leiden Institute of Chemistry
+31 71 527 4545
d.g.h.hetterscheid@chem.leidenuniv.nl
www.universiteitleiden.nl/en/science/chemistry
Please note, this article will also appear in the first edition of our brand new quarterly publication. Subscribe here for all the exciting news updates.