Joey Shapiro Key, an Assistant Professor at University of Washington Bothell, looks at the advent of multi-messenger astronomy and the role that gravitational wave observatories will now play.
The first incident detection of gravitational waves by the twin Laser Interferometer Gravitational wave Observatory (LIGO) detectors in 20151 and the first multi-messenger detection of electromagnetic and gravitational waves from colliding neutron stars in 20172 ushered in a new era for discoveries in astrophysics and cosmology.
The first handful of gravitational wave detections came from the merger of stellar mass black holes in distant galaxies, followed by the stunning observation of a binary neutron star collision and the associated gamma ray burst and brief brightening known as a kilonova. Other predicted sources of gravitational waves detectable by LIGO include nearby supernovae, rapidly spinning neutron stars, a gravitational wave background, or a network of cosmic strings.
Reaching beyond the predicted physics, there is great potential in gravitational wave astronomy to discover new and unknown phenomena through their gravitational wave emission. The potential discovery space in gravitational wave astronomy relies on our to understand our current and future detectors and to maximise our sensitivity to signals from unexpected sources of gravitational waves. This is an exciting time as we confront the limits of our observations and push into the unknown, bringing the next revolution in our understanding of the cosmos.
The spectrum of gravitational waves
LIGO partners with the Virgo observatory with sensitivity to events at the highest gravitational wave frequencies, while new gravitational wave observatories will extend our ability to reach across the gravitational wave spectrum (see Fig. 1).
Just as we observe different astrophysical phenomena at different wavelengths of light, the gravitational wave spectrum also sees radically different skies at different wavelengths. Astronomers have been using electromagnetic observations for hundreds of years to understand the cosmos, while observational gravitational wave astronomy has been a reality only since 2015. Maximising the science capabilities for gravitational wave observations requires improvements to detector technologies as well as a new set of data science techniques.
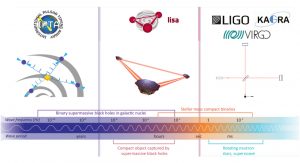
© LIGOSonoma State UniversityA Simonnet
A global network of gravitational wave detectors
On 14 September 2015 the two LIGO detectors registered the gravitational wave signal GW150914, created by the cataclysmic merger of two black holes in a distant galaxy more than one billion light years from the Earth. This marked the beginning of the era of gravitational wave astronomy and the 2017 Nobel Prize in Physics was awarded for the groundbreaking discovery.
On 17 August 2017, astronomers around the world were alerted to the gravitational wave event GW170817, observed by the LIGO and Virgo detector network. Less than two seconds after the GW170817 signal, NASA’s Fermi satellite observed an associated gamma ray burst, and within minutes telescopes around the world began an extensive observing campaign to identify the host galaxy and observe it with instruments sensitive across the electromagnetic spectrum. GW170817 marked a new era of multi-messenger astronomy, where for the first time the same event was observed by both gravitational waves and electromagnetic waves, providing a global picture of the physics of a cataclysmic event.
The first detections of gravitational waves by LIGO and Virgo make abundantly clear the necessity for robust, comprehensive data analysis methods in order to identify, characterise, and distribute timely alerts for gravitational wave signals. The identification of marginal signals buried in the detector noise and the search for unexpected gravitational wave signals holds the potential for the next great discovery.
The International Pulsar Timing Array (IPTA)
Nature has provided us with a galactic-scale gravitational wave observatory in the form of millisecond pulsars in the Milky Way galaxy. Millisecond pulsars are rapidly-rotating neutron stars that emit a narrow beam of radio waves that can be observed by radio telescope on Earth. Since gravitational waves stretch and shrink the fabric of spacetime, the distances between radio pulses from pulsars change as gravitational waves pass through our galaxy. As space stretches, some pulses arrive later than expected, while as space shrinks, some pulses arrive sooner than expected. A pulsar timing array (PTA) uses radio telescopes to precisely monitor the pulse arrival times of millisecond pulsar signals in order to observe a coherent shift due to gravitational waves.
The North American Nanohertz Observatory for Gravitational waves (NANOGrav) is a National Science Foundation (NSF) Physics Frontiers Center (PFC) observing millisecond pulsars to search for gravitational waves. Together with the European Pulsar Timing Array (EPTA) and Parkes Pulsar Timing Array (PPTA) these collaborations form the International Pulsar Timing Array (IPTA), combining data and expertise to maximise our sensitivity to nanohertz gravitational waves.3 New observing and analysis techniques are developed at the interface between radio astronomy and gravitational wave astronomy to increase our sensitivity in the nanohertz region of the gravitational wave spectrum where we will observe the signatures of supermassive black hole binary systems and study the formation and dynamics of galaxies.
The Laser Interferometer Space Antenna (LISA)
The Laser Interferometer Space Antenna (LISA) will observe gravitational waves from space starting in the 2030s.4 LISA will be sensitive to a portion of the gravitational wave spectrum that will be plentiful with gravitational wave signals, presenting us with unique data science challenges (see Fig. 2). Unlike the gravitational waves detected thus far by LIGO and Virgo, sources observed by LISA will be long-lasting, spanning several weeks to the entire mission duration. LISA presents a unique opportunity to provide advanced warning to electromagnetic astronomers, with time to co-ordinate multi-messenger observing campaigns of cataclysmic events in the distant Universe.
The persistence of the sources detectable by LISA present a new data science challenge for gravitational wave observers, who will now have to contend with the changing behaviour of the detectors and many overlapping signals simultaneously present in the data. Like trying to isolate a single conversation at a crowded party, individual gravitational wave signals will have to be separated and characterised to accurately identify and localise the source for further study.
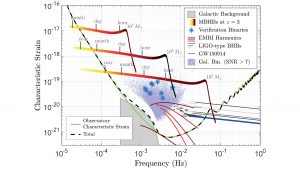
© LISA Consortium
The future of gravitational wave astronomy
The discovery of merging black holes in 2015 by LIGO forever shifted the landscape of observational astronomy, and the incredibly rich science yield from the joint gravitational wave and electromagnetic observations of merging neutron stars in 2017 profoundly demonstrated how this new observational capability benefits the broad scientific community. The discoveries were made possible by the development of incredible detector technologies, and critical advancements in data science which have matured along with the observatories’ capabilities.
We are entering the era of routine observations of astrophysical systems through their gravitational wave emission. The LIGO and Virgo detectors have been joined by the Japanese detector KAGRA, and will eventually be accompanied by a third LIGO detector located in India to form a detector network spanning the globe. With advances in modern data science we are poised to realise the great potential payoff for detections of new types of gravitational wave sources.
As our observing window into the gravitational Universe broadens, new data science challenges will be confronted, and overcome, to continue expanding our knowledge of the dynamic Universe. Future generations of scientists will routinely use gravitational wave observations, along with more traditional observing techniques, to unveil and understand new phenomena in astrophysics, cosmology, and fundamental physics.
Acknowledgements
The author is supported by NSF CAREER award 1944412. This article is adapted from Gravitational Wave Astronomy in American Scientist (Key & Littenberg, Sept 2018)5 with permission of the authors.
References
- LIGO Scientific Collaboration and Virgo Collaboration, ‘Observation of Gravitational Waves from a Binary Black Hole Merger’, Physical Review Letters, 116, 061102 (2016)
- LIGO Scientific Collaboration, Virgo Collaboration, and multi-messenger partners, ‘Multi-Messenger Observations of a Binary Neutron Star Merger’, Astrophysical Journal Letters, Volume 848, Issue 2 (2017)
- Perera, B. B. P., et al, ‘The International Pulsar Timing Array: second data release’, MNRAS 490 4666-4687, 2019
- K. Danzmann et al, ‘A proposal in response to the ESA call for L3 mission concepts’, arxiv.org:1702.00786, 2017
- J.S. Key and T.B. Littenberg, ‘Gravitational Wave Astronomy’, American Scientist, 106 (5): 276, 2018
Joey Shapiro Key
University of Washington Bothell
+1 425 352 5497
joeykey@uw.edu
https://sites.uw.edu/joeykey
Please note, this article will also appear in the third edition of our new quarterly publication.