Professors Philip L Cole (Lamar University), Kyungseon Joo (University of Connecticut), and Hartmut Schmieden (University of Bonn) are exploring the properties of subatomic matter through nucleon excitations. They discuss their complementary studies with CLAS12 at Jefferson Lab (JLab) and with BGOOD at the Electron Stretcher Accelerator (ELSA) in Bonn.
Nuclear and particle physics have revealed the structure of the matter surrounding us. Matter consists of atoms composed of a diffuse cloud of electrons surrounding a densely packed nucleus. Atoms can be rendered visible through special microscopy, even though they are only 10–10 m across, or 1/10 of a nanometer. On the scale of the atom, the nucleus is but a tiny speck of matter. It is 100,000 times smaller than the diameter of the electron cloud, and yet it contains 99.95% of the entire mass of the atom. The nucleus itself is a composite object, made up of protons and neutrons, generically termed ‘nucleons’. A nucleon can be probed through electromagnetic interactions by the scattering of high-energy electrons. Such experiments revealed a substructure inside the nucleons, termed quarks.
Probing the excited states of the proton
Associated with the composite nature of the nucleon is a rich spectrum of excitations. These excited states are called ‘nucleon resonances’ (N*s) and are short lived, on the order of a trillionth of a trillionth of a second (10−24 s).
The primary research question that the transatlantic BGOOD and CLAS12 experiments seek to address is: what are the internal mechanisms of how quarks bind together in creating these excitations?
Until recently, the structure of bound quark states (generically termed hadrons) was thought to be formed of either 3-quark configurations for nucleon-type objects (called baryons) or 2-quark (or more precisely, quark-antiquark) compositions for entities labelled mesons. This picture is the essence of the Quark Model. There are six types or flavours of quarks, in order of mass: up, down, strange, charm, bottom, and top (u,d,s,c,b,t).
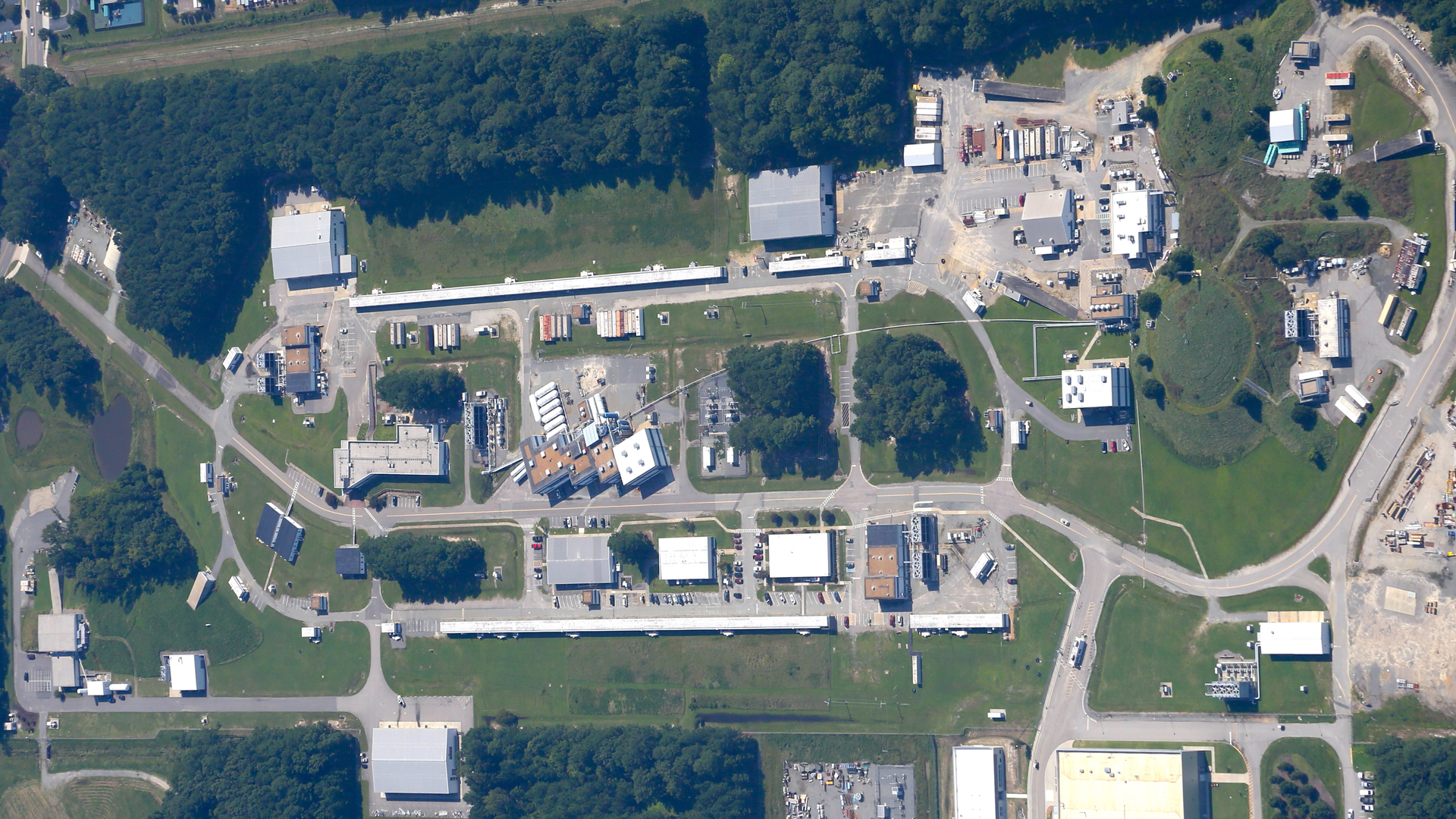
New experiments in the sector of c-quarks indicate that excited structures may appear beyond the description of the Quark Model, in particular mesons containing four quarks, and baryons composed of five quarks. Recent developments in theoretical physics suggest those might be realised through a type of binding similar to molecules formed from atoms, albeit on a vastly smaller scale and based upon the vastly more forceful strong interaction. Such objects would result, for example, from the binding together of a baryon-type with a meson-type configuration, similar to the mutual binding of nucleons inside a nucleus rather than the binding of quarks inside a nucleon. Corresponding structures may appear exotic in the light of the Quark Model, but not so from the perspective of Quantum Chromodynamics (QCD), the theory of strong interactions.
The issue is whether such structures are exclusive to charm and heavier quarks or may further represent a more general feature of composite structures in the strong interaction regime and thereby be manifested in the sector of the light u, d, and s quarks. CLAS12 and BGOOD are sensitive to these three quark flavours. A common goal of both experiments is to disentangle the spectrum, and to understand structure and hence the effective degrees of freedom of individual baryonic excitations. The common tool is to use energetic gamma-ray photons produced from high-energy electron beams. CLAS12 further makes use of the direct scattering of electrons off nucleons for resonance excitation studies, employing the same method used in the discovery of quarks.
The role of CLAS12
The CEBAF Large Acceptance Spectrometer (CLAS12)1 recently came online for operation in Hall B at Jefferson Lab (JLab). The CLAS12 set of detectors is designed to study electro-induced nuclear and hadronic reactions. This spectrometer provides efficient detection of charged and neutral particles over a large fraction of the full solid angle. CLAS12 was built as part of the energy-doubling project of Jefferson Lab’s Continuous Electron Beam Accelerator Facility (CEBAF), funded by the United States Department of Energy.
The CEBAF accelerator was originally designed to provide electrons at energies of four billion electron-volts, or 4 GeV, but efficiencies in its design and extensive experience in running the facility allowed JLab operators to achieve 6 GeV electron beams, beyond its original design configuration. The $338m, multi-year 12 GeV CEBAF Upgrade Project tripled CEBAF’s original operating energy. CEBAF can now deliver its full-energy electron beams, up to 12 GeV, to the Hall D complex, and up to 11 GeV electron beams into its original experimental areas, Halls A, B, and C. (See Fig. 1).
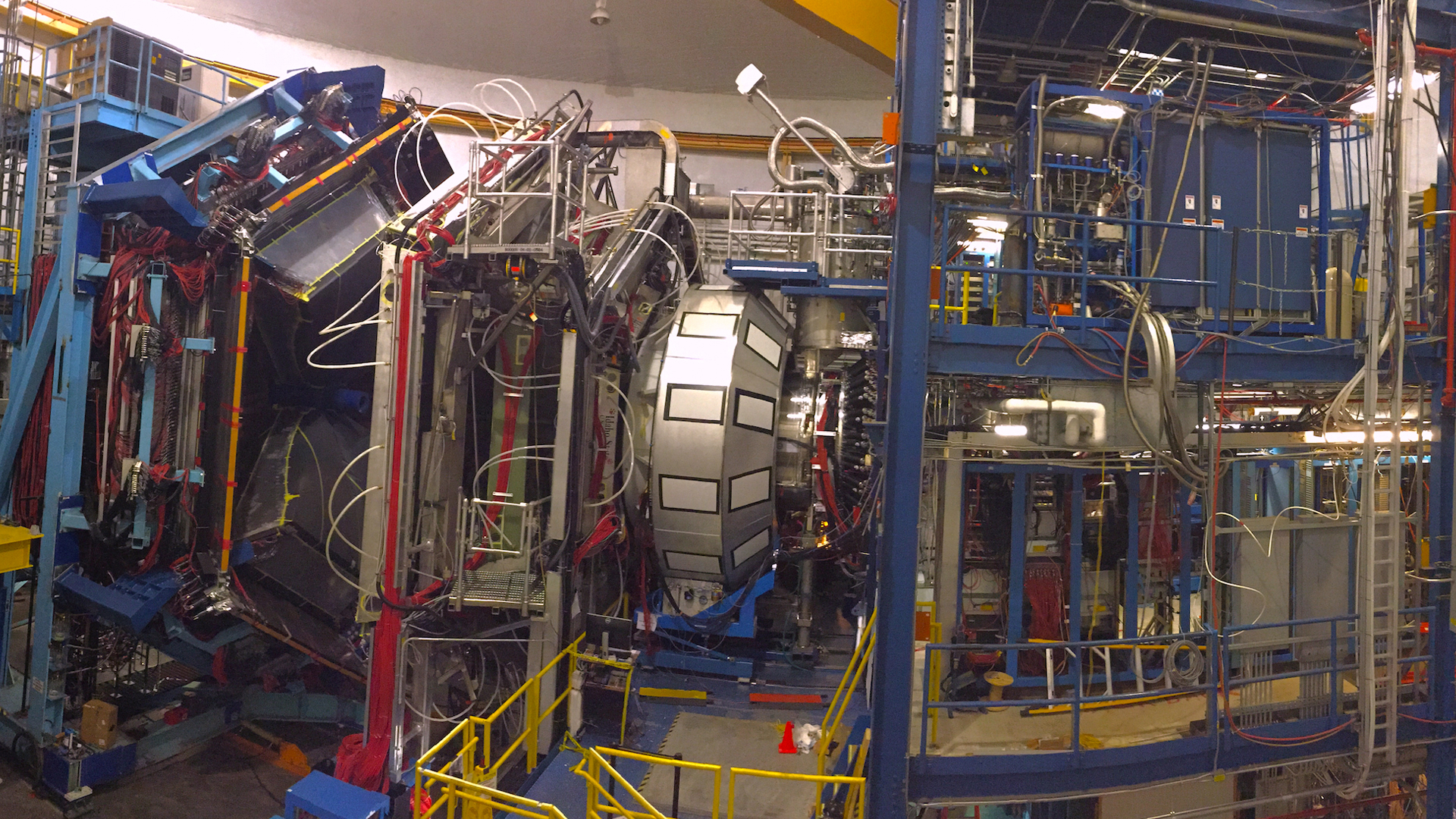
An international collaboration of 48 institutions contributed to the design and construction of the detector hardware, developed the software packages for the simulation of complex event patterns, and commissioned the detector systems. CLAS12 (see Fig. 2) is based on a dual-magnet system with a superconducting torus magnet that provides a largely azimuthal field distribution that covers the forward polar angle range up to 35°, and a solenoid magnet and detector covering the polar angles from 35° to 125° with full azimuthal coverage. Trajectory reconstruction in the forward direction using drift chambers and in the central direction using a vertex tracker results in momentum resolutions to within 1% and 3%, respectively. Cherenkov counters, time-of-flight scintillators, and electromagnetic calorimeters provide excellent particle identification. Fast triggering and high data-acquisition rates allow operation at a luminosity of 1035cm−2s−1. These capabilities are being employed in a broad program to study the structure and interactions of nucleons, nuclei, and mesons, using polarised and unpolarised electron beams and targets for beam energies up to 11 GeV.
A cornerstone of the CLAS12 science programme is in the spatial studies of the structure of excited nucleon states.2 The scattering of electrons off nucleons allows for ascertaining subnuclear length scales. Such a precise probe represents the only source of spatial information for many facets in the emergence of nucleons from their underlying substructure. The CLAS12 N* programme will allow us to probe the dressed quark mass function over a wide range of distance scales. In this kinematic range, the N* studies will probe distances spanning the transition between the almost bare and massless QCD-quarks to the fully dressed constituent quarks of ≈400 MeV mass. Such measurements will ultimately address the open question in QCD of where over 98% of the hadron’s mass comes from. Analyses of the CLAS12 N* results will therefore shed light on the transition between the core of three confined quarks and the cloud of hadrons in N* structure.
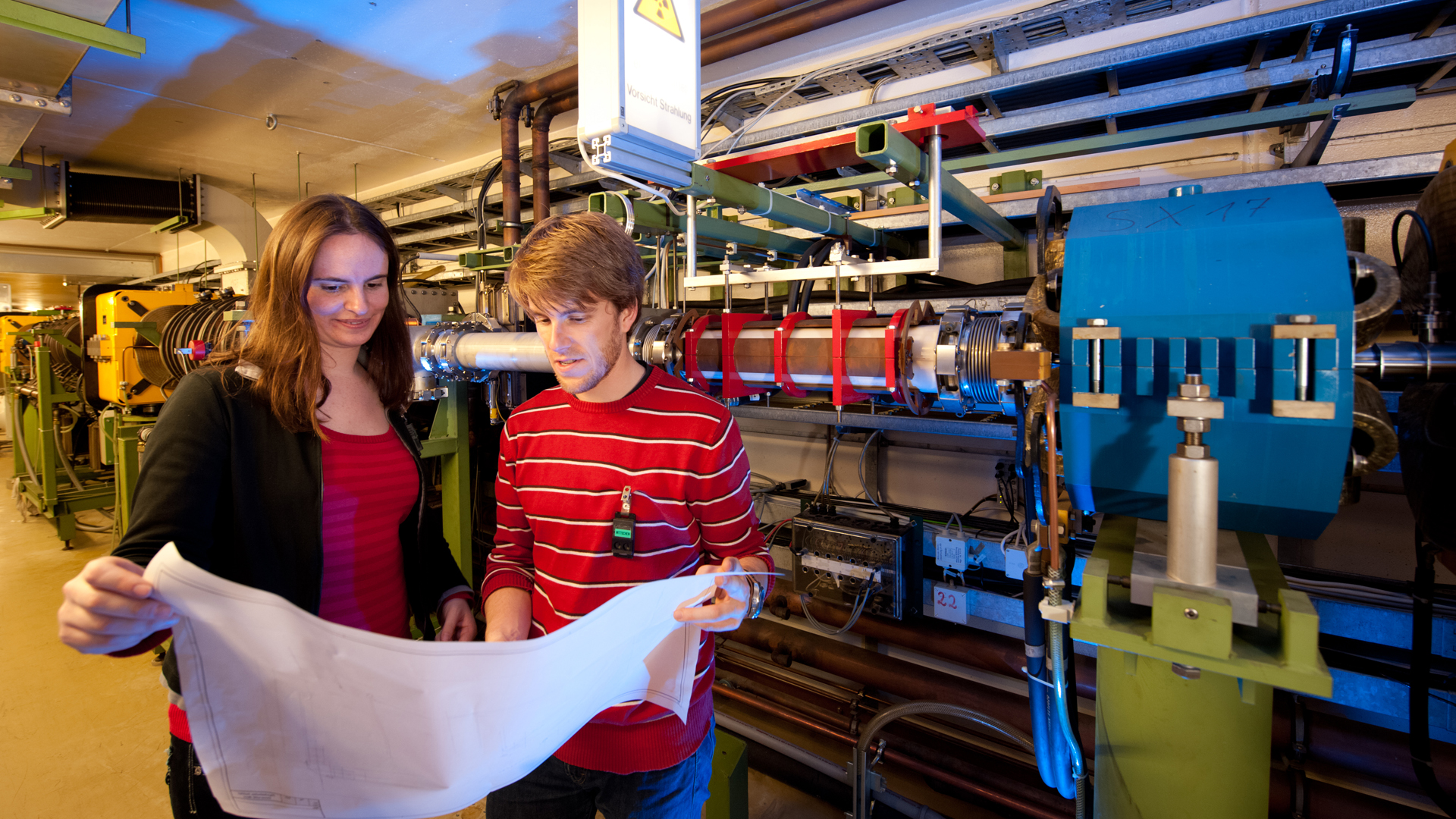
© Volker Lannert/University of Bonn
The role of BGOOD
The BGOOD experiment is situated at the ELectron Stretcher Accelerator ELSA3 at the Rheinische Friedrich-Wilhelms-Universität Bonn, Germany, which is in contrast to the national large-scale facility at JLab. BGOOD and ELSA provide a university environment for the outstanding education of physics students and junior researchers. Fig. 3 shows a team of students working at the accelerator.
The BGOOD experiment is carried out by an international collaboration, with members and contributions from Germany (University of Bonn), Italy (Universities and INFN Pavia, Roma ‘Tor Vergata’, Torino, Roma ‘La Sapienza’ and Istituto Superiore di Sanità, Frascati, Catania, Messina), Russia (St. Petersburg and Moscow), Ukraine (Kiev), and one of the co-authors (PLC) is from the sole American Institution in the BGOOD collaboration.
The ELSA electron beam of up to 3.2 GeV energy is converted into an energy-tagged photon beam, impinging upon a fixed proton or deuteron (for studies of reactions off the neutron) target. BGOOD occupies a complementary experimental space between CLAS (the predecessor of CLAS12) to CLAS12. It is tailored to accurately identify and measure charged particle momenta at extremely forward directions to within one degree from the incident beam, which directly has to pass through these forward detectors. This kinematic acceptance ensures that only minimum momentum needs to be taken by recoiling hadronic systems, permitting the formation of possible loosely bound molecular-type configurations. The detector setup comprises a forward magnetic spectrometer centered around an open dipole (OD) magnet of 100-ton weight. Trajectory reconstruction using scintillating fibre and drift chamber detectors results in a momentum resolution of 3%. The forward spectrometer is complemented with a central BGO calorimeter hermetically enclosing the target. Bismuth germanate or BGO (Bi4Ge3O12) are scintillating anorganic crystals that are sensitive to neutral and charged particle detection.
The two main components BGO and OD were eponymous for the experiment, hence the name BGOOD. Details of setup and performance are described in the technical paper.4 Fig. 4 shows the experimental area.
One central issue of investigation at BGOOD is the enigmatic Λ(1405) baryon. Recent results clearly confirm experiments of CLAS on its unusual structure in the perspective of the quark model. Further interesting threshold effects in the s-quark sector have already been measured at BGOOD and preliminary results reported.5 One feature is a cusp-like drop in strength in the very forward K+Σ0 photoproduction off protons, a reaction involving u, d, and s quarks. A second one concerns an predicted enhancement in the K0Σ0 channel on the neutron, which was predicted in theoretical models in analogy to the pentaquark Pc states discovered at LHCb in the c-quark sector.6 This may all be consistent with the picture of quasi-molecular structures to also exist in the uds-quark sector.
The path forward
There is, without a doubt, much more to the physics of nucleon excitations beyond the static Quark Model. BGOOD and CLAS12 are complementary experiments. Both seek to elucidate the pattern of baryonic excitations, from which the effective underlying degrees of freedom can be determined. And, in turn, CLAS12 has the added benefit of being able to ascertain the spatial substructure of resonances over a wide range of length scales.
At the time of their discovery, the multi-quark structures in the c-quark sector seemed highly exotic. This formation beyond the 3-quark state may very well turn out to be quite common in the realm of strong interactions. Together, through our transatlantic endeavours we seek to help unravel this puzzle of how quarks self-assemble in nucleon excitations.
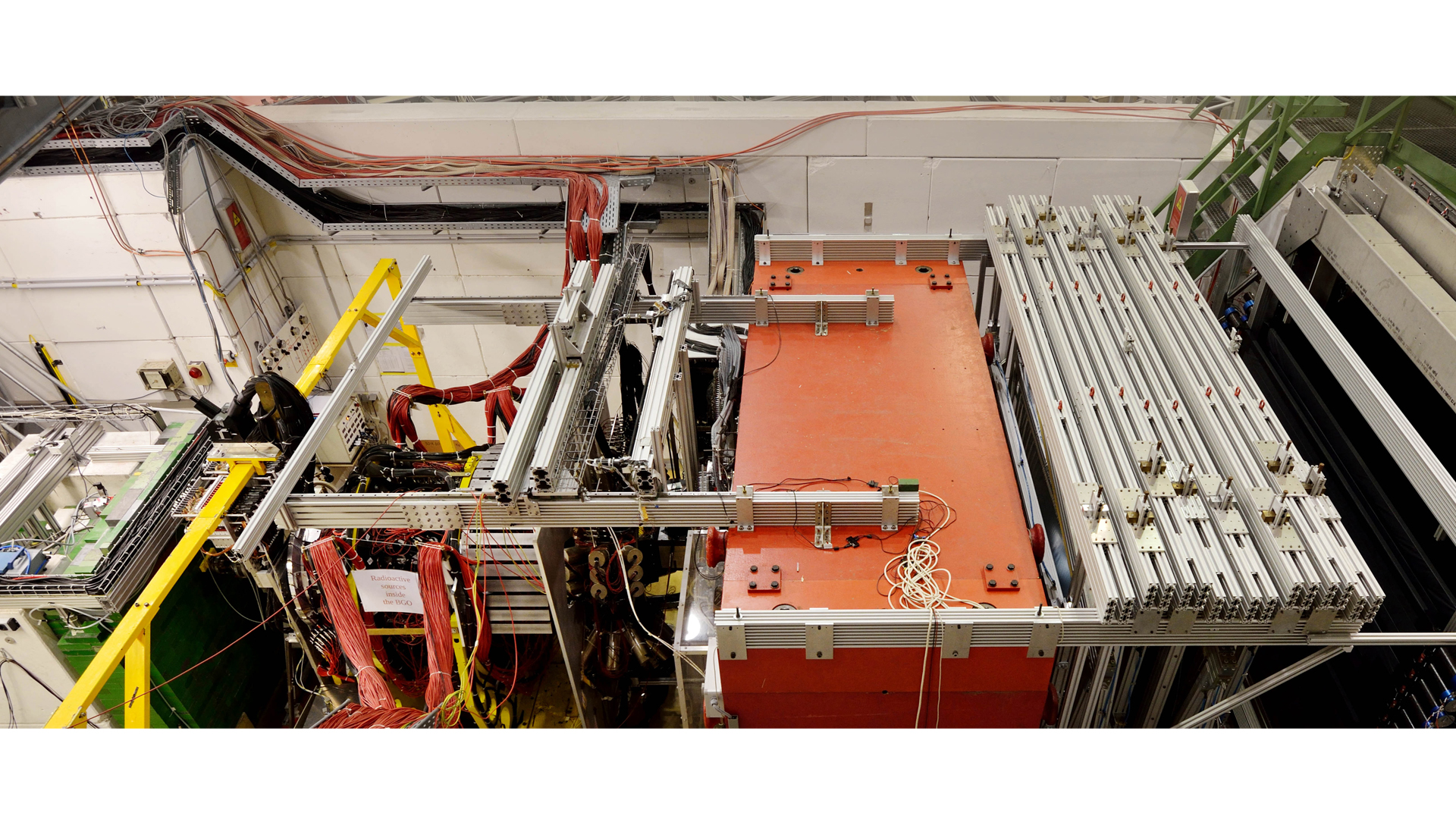
© BGOOD collaboration
Acknowledgements
The Jefferson Science Associates, LLC, manages and operates the Thomas Jefferson National Accelerator Facility for the US Department of Energy (DOE) under Contract No. DE-AC05-06OR23177. Professor Kyungseon Joo is funded by DOE Grant No. DE-FG02-04ER41309 and Professor Cole is funded through the National Science Foundation (PHY-2012826). Both Professor Cole7 and Professor Joo8 were also Fulbright Scholars at separate European Nuclear Physics Laboratories.
BGOOD has benefitted from support of all involved institutions. Its realisation would not have been possible without the longstanding significant support of INFN in Italy, and the DFG (SFB-TR16, PN 388979758 and 405882627) and the federal state of North-Rhine Westphalia in Germany. The international scientific exchange is fostered through the FTD-Hadron transnational access project of STRONG-2020 within the EU’s research and innovation programme Horizon 2020.
References
- V.D. Burkert et al. (CLAS Collaboration), Nucl. Instrum. and Meth. A 959, 163419 (2020)
- I. G. Aznauryan et al., Int. J. Mod. Phys. E, 22, 6 (2013)
- W. Hillert et al., EPJ Web Conf. 134 (2017) 05002
- S. Alef et al. (BGOOD Collaboration), Eur. Phys. J. A 56 (2020) 104
- T. C. Jude et al. (BGOOD Collaboration), EPJ Web Conf. 241 (2020) 01008, and T.C. Jude et al. (BGOOD Collaboration), https://arxiv.org/abs/2006.12437v1
- A. Ramos and E. Oset, Phys. Lett. B 727 (2013) 287
- The German-American Fulbright Commission: Physikalisches Institut, Universität Bonn (2014/2015) in Germany. Fellowship at the University of Bonn: Wanderjahr, Wunderjahr, Wendejahr
- The Franco-American Fulbright Commission: Fellowship at Institut de Physique Nucléaire d’Orsay (2015/2016) in France
Please note, this article will also appear in the sixth edition of our quarterly publication.