Professor Philip L Cole, of Lamar University, discusses the approved experiment E45 at J-PARC that will make use of high-intensity pion beams to study the inner structure of protons in the second and third resonance regions.
Professor Philip L Cole is an experimental nuclear physicist and Chair of the Department of Physics at Lamar University. Joining Lamar in July 2017, Cole conducts his research at Jefferson Lab (CLAS12 and GlueX) in Newport News, Virginia, and at ELSA (BGOOD) at the University of Bonn.
Located in Beaumont, Texas, Lamar University is the largest university in the heart of the region known as the ‘Golden Triangle’ (Beaumont/Port Arthur/Orange). The primarily undergraduate institution is a culturally diverse, state-funded, Carnegie-recognised university enrolling around 15,500 students, predominantly from Southeast Texas. Located amongst a large oil infrastructure, Lamar is well-positioned to focus on engineering and physical sciences.
Professor Cole has established a reputation within the nuclear and particle physics community for a variety of past observations, including his Transatlantic research work, in collaboration with Professor Kyungseon Joo (University of Connecticut) and Professor Hartmut Schmieden (University of Bonn), exploring the properties of subatomic matter through the excited state of protons.
More recently, Professor Cole has been appointed as co-spokesperson for the approved experiment E45 at the Japan Proton Accelerator Research Complex (J-PARC) high-intensity proton accelerator facility. This Transpacific collaboration will make use of the high-intensity proton beams on the Tokai campus of the Japan Atomic Energy Agency will use the complementary tool of pion beams to study the inner structure of protons.
Professor Cole spoke to Innovation News Network to explain the collaboration in further detail.
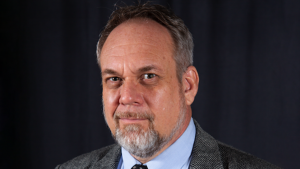
Can you outline your role as the co-spokesperson for the J-PARC experiment?
For a full study of excited baryons, we need both electron/photon beams (Germany and the US) and pion beams (Japan and Germany). I am positioned right at the intersection of these studies. In May 2017, we held the ECT* workshop, Space-like and Time-like Electromagnetic Baryonic Transitions, which convened in Trento, Italy, at the European Centre for Theoretical Studies in Nuclear Physics and Related Areas (ECT*). I organised the workshop, alongside time-like nuclear experimentalist Dr Béatrice Ramstein, from the Institut de Physique Nucléaire d’Orsay, and nuclear theorist Dr Andrey Sarantsev, of the University of Bonn and the Petersburg Nuclear Physics Institute in Gatchina, Russia. I represented the space-like community, focussing on the use of electron beams, whilst Dr Ramstein’s work centres on the baryon transitions with pion beams. Broadly speaking, space-like is related to the production of the excited baryons, whereas time-like involves the subsequent decay of those baryon resonances.
To our knowledge, this was the first time the time-like and space-like communities held a joint workshop on baryon resonances. Around 30 nuclear physicists from across Asia, Europe, and North America attended this five-day workshop, where participants were split evenly between the respective time-like and space-like communities of experimentalists and theorists. I delivered the concluding talk, titled ‘Future directions of space-like and time-like baryonic form factors’. We have since convened a few plenary talks, as well as parallel sessions, at the last two biennial NSTAR set of workshops. The next International Workshop on the Physics of Excited Nucleons (NSTAR 2022) will convene in Santa Margherita, Italy, in October 2022. NSTAR are the focus meetings for nuclear physicists active in excited baryon research.
In 2012, the experimental proposal for the J-PARC 50-GeV Proton Synchrotron, 3-Body Hadronic Reactions for New Aspects of Baryon Spectroscopy, was approved for 15 days of running time. The actual beamtime will be determined before the running of the experiment, which is officially known as E45. The spokespeople were Dr Ken Hicks, from Ohio University, and Dr Hiroyuki Sako, of Japan Atomic Energy Agency (JAEA). Incidentally, Dr Sako also attended the ECT* workshop in Trento. Due to Dr Hicks’ recent appointment as a Program Manager at the U.S. Department of Energy, he resigned from the E45 collaboration to avoid a conflict of interest.
Following Dr Hicks’ departure, I was elected by my colleagues as the co-spokesperson of the E45 experiment in September 2021, responsible for leading the US side of the collaboration. This will include ensuring all the necessary hardware and software on our side is ready, as well as seeking funding for the necessary travel for staffing the shifts and human resources for analysing the data. We expect many PhD topics and research opportunities for scientists early in their careers.
On 20 January 2022, Dr Sako presented our beamtime request to the J-PARC Program Advisory Committee. We expect to run the experiment in 2025 but we are still awaiting confirmation from the J-PARC management on the exact schedule.
Can you explain the basis of the experiment? What are your main objectives currently and how do you set out to achieve these?
Our knowledge of how protons and neutrons are constructed from their quark and gluon constituents is rudimentary at best. Understanding exactly how the strong force is generated by the exchange of field carriers inside the nucleus is one of the greatest intellectual challenges facing nuclear physicists today. Indeed, the 2004 Nobel Prize in Physics was awarded for the discovery of asymptotic freedom – the prediction that the force between valence quarks decreases as quarks get closer together. In contrast, the force becomes immense as quarks are separated, creating intense fields. As a result, the nucleon mass is not simply a sum of three valence quark masses but is primarily due to the energy contained within the strong field binding those quarks inside the nucleon. We now know that nucleons are formed of three valence quarks, bound in an effervescent sea of self-interacting gluons and quark-antiquark pairs that fluctuate into and out of existence. It is through this mechanism that the valence quarks become dressed and thereby the nucleon acquires its mass.
An energetic particle, such as an electron or pion, incident on a nucleon can interact directly with one of the valence quarks inside, causing the quark to undergo a flip in spin or endowing the quark with an orbital or radial excitation. With a quark in a higher energy state, the excited nucleon becomes more massive. These excited states are called ‘nucleon resonances’ (N*s) and are short lived, on the order of 10−24 s. These excited nucleons will dominantly decay into a ground-state nucleon, producing other strongly interacting particles, known as mesons. A pi meson or pion (π) is the lightest member of the meson family, possessing roughly 15% the mass of the proton. The types of mesons produced and how they are distributed in the phase space of the decay process provides key information on the internal symmetries of the quarks in the nucleon. The study of these excited states is known as ‘spectroscopy’. Just as ordinary optical spectroscopy proved to be the incisive tool for understanding the electronic structure of the elements, we expect that, by analysing the structure of the excited baryons, we can learn how baryons emerge from quarks and gluons. Quantum chromodynamics (QCD), the theory that governs the strong force, will be our guide.
The nucleon resonance spectrum holds fundamental information about nonperturbative QCD. However, the resonance spectrum is not easily extracted from experimental data, due to the large widths of the overlapping resonances. Precise data on both electro-/photoproduction and pionic reactions are necessary to separate the nucleon resonances using a partial-wave analysis (PWA). PWA is a mathematical means for extracting the quantum numbers of spin and parity of the underlying N*s. For PWA to work efficently, complete and accurate data from both pion and electron beams are required to constrain all the overlapping N* amplitudes over a broad range of energy. The coordinated study of the reactions πN → ππN and γp → π+π−p, for instance, is what is meant by the coupled-channels approach. Recent theoretical advances have shown the importance of coupled-channels effects in a PWA, and that it is necessary to complement the high-quality data on the πN → πN channel with similar-quality data for the πN → ππN and πN → KY reactions. The latter two reactions were measured with only modest precision about four decades ago. Here, K is a meson formed of a strange/anti-strange quark pair and Y is a hyperon, akin to a nucleon, but formed with a strange quark replacing one of the three up or down quarks. With J-PARC, it is now possible to make precise measurements of these reactions, and hence extract the nucleon resonance spectrum.
One exciting development comes from recent lattice QCD calculations, which predict that hybrid baryons (with gluonic excitations in the three-quark system) should exist. If hybrid resonances exist, it is essential to get hadronic data on πN → ππN to use in dynamical coupled-channels PWA that provide the best chance of reliable resonance extraction from the data. Preliminary indications from photoproduction data at CLAS and BGOOD suggest that an extra resonance, in addition to the known quark-model resonances, has been seen in the reaction γp → π+π−p at a mass close to that predicted from the lattice for a hybrid baryon. Whether this is a regular baryon resonance or a hybrid baryon resonance, precise hadronic data are needed to untangle it from other nearby (overlapping) baryon resonances having large widths. We propose to measure the πN → ππN and πN → KY reactions at J-PARC with sufficient precision to search for baryon resonances.
The proposed experiment will be carried out on the K1.8 beamline of the Hadron Hall of the J-PARC accelerator. The primary goal is to measure the πN → ππN reaction, and, in particular, to search for evidence of new baryon resonances through a dynamical coupled-channels PWA. A secondary goal is to gather data on the πN → KY reaction, which is needed because very little is known experimentally about N* resonances that couple to KY decay, and because the K+Σ+ final state is key to analyses of ∆* resonances, which decay into purely I = 3/2 channels. The high-statistics photo- and electroproduction data from Jefferson Lab makes it even more compelling to get high-statistics hadronic data. Without hadronic data, a coupled-channels partial-wave analysis to search for new nucleon resonances is not sufficiently constrained. The simultaneous analysis (Transpacific and Transatlantic) of both the hadronic and photoproduction data, will give that powerful constraint on the PWA.
How long is the experiment expected to last for and what resources will be required?
We have requested 15 days of beamtime or 45 shifts, with seven days for set-up. The cross section for pion-induced reactions is at least 100x greater than that for electroproduction of the double-pion channel. This means that such pion beamtime is indeed deeply significant and will provide that missing link for our coupled-channel analyses in resolving the structure and nature of the excited states of the nucleon.
The range of the energy for the E45 experiment is 1.50 – 2.15 GeV (p = 0.73 – 2.0 GeV/c). By way of comparison, the mass of the proton is 0.94 GeV/c2. E45 will be a thorough systematic study with the fine granularity of the π− beam having 24 (energy) x 20 (angle) bins and similarly for the π+ beam: 23 (energy) x 20 (angle) bins. The expected number of events/bin will be 32,000. This means we will accumulate 30M events in the span of 15 days. E45 will significantly enhance the world’s ππN data of 0.24M events by at least a factor of 130.
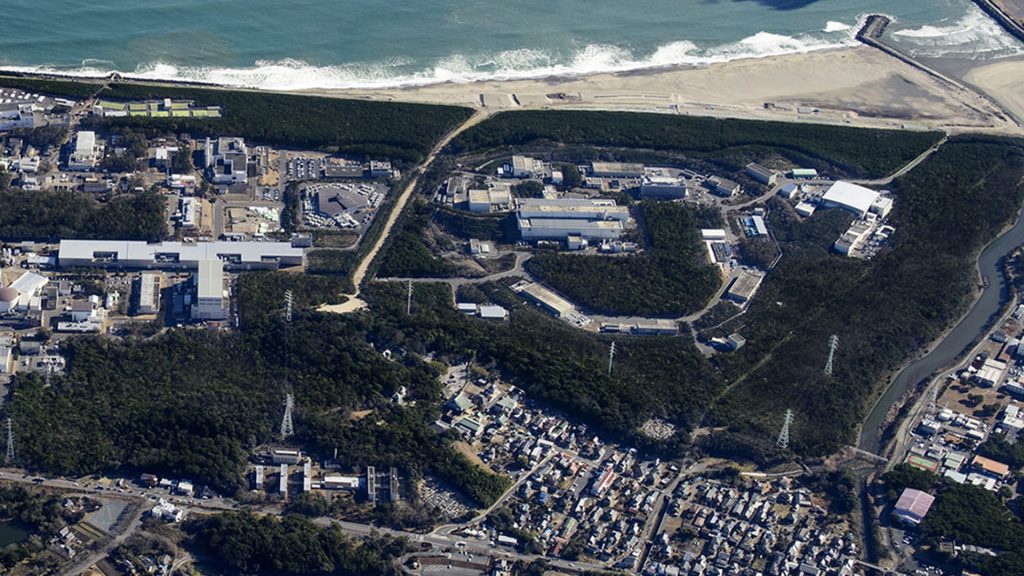
What are the current challenges that your team is facing? What can be done/has been done to overcome these challenges?
We are on target and do not expect any untoward challenges in terms of hardware and data acquisition. All the necessary equipment is ready and we now need to coordinate resources and manpower for understanding the PWA in the time-like regime. The experimental group HADES at GSI has paved the way in such PWA calculations, in coordination with the Bonn-Gatchina group led by Dr Andrey Sarantsev, who also co-organised our inaugural ECT* workshop back in 2017. The other co-organiser, Dr Béatrice Ramstein, is a key member of HADES. We have been formulating how we can coordinate PWA resources in the United States (JLab), Germany (HADES and Bonn/Gatchina), and Japan (JAEA). Community interest is strong and this will enable us, for the first time, to coordinate the resources of the space-like and time-like communities to work on an overlapping project.
What insights are you hoping to be gained from the experiment? What impact will the findings of the experiment have on future innovation and research?
With a modest beam-time request, an entire generation of data from the 1970s and 1980s for the πN → ππN and πN → KY reactions can be remeasured with much higher statistical and systematic accuracy and precision. Because these data are necessary to the dynamical coupled-channels calculations that are now available to deduce the nucleon resonance spectrum, this is a golden opportunity for resolving the conundrum of the proton. J-PARC is the only facility in the world at present that can measure these important data with both π+ and π− beams over the entire second and third resonance regions. We are collaborating with the experimental collaboration HADES, who will have overlap using the GSI/FAIR facility in Darmstadt, Germany. We also wish to point out the recent paper from HADES using a π− beam in the second resonance region.
Through this broad and collaborative international effort, our team will be poised to forge an understanding of the essentially nonperturbative features of the Standard Model. It offers the possibility of answering the three fundamental questions at the heart of hadronic physics:
1) What is the essence of confinement? That is, why is it that the three valence quarks are bound together so tightly and how does their binding evolve for nucleon resonances having different quantum numbers? And what role does the meson-baryon cloud play in quark-gluon confinement?
2) How is confinement connected with dynamical chiral symmetry breaking, which accounts for the origin of roughly 98% of the visible mass in the Universe? The Higgs mechanism accounts for only 2% of the mass, the rest is due to the strong field in the nucleon.
3) Can the Standard Model be further developed to successfully describe the complex structure of all excited baryon states and, if so, how does the spectrum of baryons emerge from the strong interaction among dressed quarks and gluons? How may we improve the connection between ab-initio calculations of QCD and the experimental observables?
Everything is coming together now. With all the new experiments using photon, electron, and pion beams scheduled to take place in the near future (or are already taking place) at JLab, J-PARC, Spring-8, ELSA, GSI, FAIR, and MAMI, and with the advances of theory and phenomenology, we are on the verge of solving the fundamental problem of how structures and mass emerge from quarks and gluons. We will, however, continue to stay on that frustrating verge, unless we coordinate and consolidate our efforts across continents and across theoretical and experimental groups.
E45 and HADES serve as a catalyst to make the transformation in the time-like regime. Our experiments with pion and electron beams, along with the emerging collaborations, will ignite the spark that will catalyse a transformative understanding of how force and mass interrelate at the subatomic scale.
Acknowledgements
I gratefully acknowledge Dr Hiroyuki Sako of the Japan Atomic Energy Agency for his insights into the physics and his leadership on this experimental project.
Please note, this article will also appear in the ninth edition of our quarterly publication.