As we try to mitigate a sixth mass extinction, Professor Dr Jörns Fickel of Leibniz Institute for Zoo and Wildlife Research explains the work taking place on species’ ability to cope with environmental changes.
One of the seventeen Sustainable Development Goals (SDG) for 2030, adopted by the United Nations Member States in 2015, is called ‘Life on Land’ and is aimed at the protection, restoration and sustainable use of terrestrial ecosystems, sustainable management of forests, halting and reversing land degradation and desertification, and last but in no way least, halting the loss of biodiversity. Because that loss has even accelerated in the last decades, the term ‘sixth mass extinction’ was coined. This refers to the five mass extinction periods that Earth’s biodiversity has already faced in the past 450 million years, each of them reducing the then having existed number of species by at least two thirds.
How to halt the loss of biodiversity
A great variety of conservation measures have been proposed, including (among others) the establishment of protected areas, increased efforts to educate about the intrinsic value of biodiversity, international treaties (e.g. CITES), even giving ecosystems a monetary value based on the ‘services’ they provide. While all these measures are necessary, we focus on the biological, the species-specific side to it. Every single species existing today does so because generations of ancestors have evolved adsaptations to the ecological niche the species lives in. In sum they are called the phenotype of a species. As a species is the sum of its individuals, it is also the sum of the genetic information – called the genome (an art-word that combines gene and chromosome) – of all individuals. In mammals, the genome consists of two sets of chromosomes, with one set coming from one parent, the other one coming from the other. The sum of all genomes is something that biologists call the genepool of a species. This genepool harbours the ‘potential’ of each species to respond to, and to cope with environmental changes. However, some of these changes may come so quickly and are so severe that they exceed the ‘coping potential’ of a species. Let’s not forget that after all, the majority of species that ever existed has already gone extinct. So, the big question is (for each species separately): to what degree can the environment change, so that a species is still able to cope with it? In other words: How large is the ‘potential’?
The first step to answer this question means deciphering which genes are responsible for which particular adaptation-conferring trait. To figure this out one compares genomes of individuals of a species in the context of the species’ environments, which we do in two ways. The first one is to consider long-term evolution. In the context of reconstructions of past climatic conditions, we can then extrapolate how past conditions have shaped the current genepool of a species. Long-term evolution works through mutations that change the function of genes. However, the frequency by which mutations occur is quite low, and not all mutations will have a positive effect. The majority is actually negative (and individuals carrying them will be selected against) or neutral, so of the few mutations that occur, an even smaller proportion will have a positive (adaptive) effect. Looking at long-term evolution means looking at a large time frame (covering many, many generations), and over a large time frame selection will cause these adaptive mutations to accumulate. Now we can find them, and this is usually done by looking at species that are very closely related but live in different habitats (e.g. grassland versus rainforest). In a comparison of the genomes of these species (by comparing the order of the four building blocks of the genetic material), most parts of the genomes will not show any differences (mutations that happened in the past) between the two species. These parts both species inherited from their common ancestor. However, the regions of the genome that do not match (because they carry many mutations) are the ones we are interested in because they will likely contain the genetic information for the adaptations to the respective environment each species lives in. These types of studies are called association studies, as they associate certain genes or groups of genes with certain adaptive traits. They are either directed at single position differences between species’ genomes in either genes or their on/off-switches (called promotor), at genes under positive selection (genes that increase fitness), or at very large chunks that differ among genomes (called ‘structural variants’, or SVs) and that may shift genes or groups of genes into a different (genomic) context or remove them completely (in case of deletions).
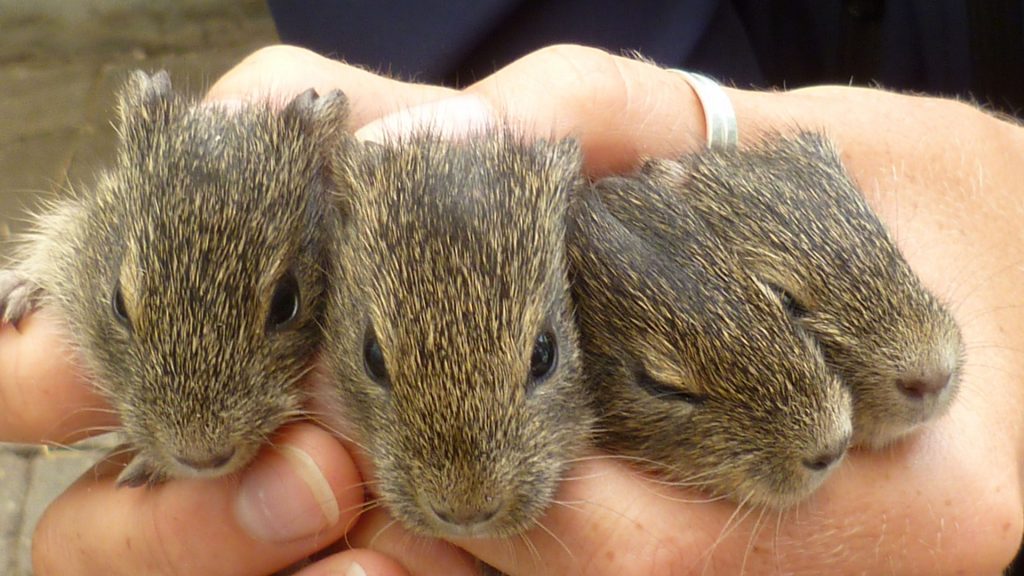
Examining reproduction and diet-related traits
The very important traits to look at with view toward future environmental changes (increased annual average temperature and shifts in vegetation) are ‘reproduction’ and ‘diet’. If the ‘reproductive potential’ of a species is negatively affected by future environmental changes, the species may go extinct. If the ‘diet utilisation potential’ is negatively affected by future environmental changes, the species may go extinct. A lot of worldwide research is directed at identifying the genes related to these traits. The species whose ‘potential’ we study are mustelids (weasels, otters, badgers, martens and the like). As mustelids live in a great variety of habitats ranging from the northern Palearctic to the Tropics, the different species live at different climatic conditions with often seasonally varying food supply (except for the Tropics). Many Northern species therefore reproduce only once a year, while tropic species reproduce more often. Our aim is to identify genes that have either been gained (e.g. additional copies via duplication) or lost (e.g. via SVs) and that appear to be associated with these traits. Using this approach, we have already identified numerous differences in the genomes of the compared species. Many of these differences affect genes whose functions are already known, allowing pinpointing them to the expression of the two traits. However, many differences we found affect genes whose functions are not (yet) known, so we do not know yet what their duplication or rearrangement means for the phenotype.
While this sounds conceptually easy, these comparisons are actually quite challenging, because analysing and comparing genomes (each of which consists of ~ 3×109 building blocks) requires both computational power and sophisticated bioinformatics. This is the reason why we participate in ERGA (European Reference Genome Atlas), a bottom-up initiative from European biodiversity researchers to sequence (at best resolution possible) all European species. In ERGA we can join forces, share resources and promote communal learning.
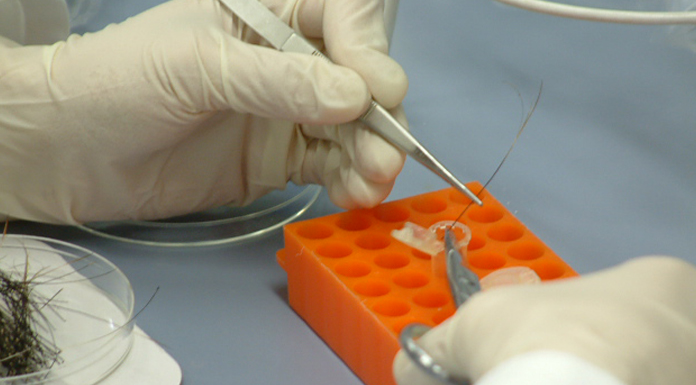
Using comparative epigenomics to study the ‘coping potential’
While the comparison of genomes from two ‘sister’ species and thereby looking at long-term evolution via mutations is one way to study the ‘coping potential’ of species, another way, but covering a much shorter period, is to use comparative epigenomics. As this approach only spans very few generations, the epigenetic ‘coping potential’ can be studied experimentally (provided the study species has a short generation time). Such experiments allow effects different environmental factors have on the genomes of species to be studied. Epigenetics does not look at mutations (which occur very infrequently, as mentioned above), epigenetics looks at modifications of an individual‘s DNA. The most common one is the enzymatic addition of a methyl-group (-CH3) to a cytosine, one of the four DNA building blocks. How does this help in studying the ‘potential’? The trick, Mother Nature has come up with, is that addition of methyl-groups to cytosines located in a promotor (the on/off switch of genes) will put that promotor in the ‘off’ position. The gene governed by this switch will now become gradually deactivated. The more cytosines methylated, the more complete the deactivation. This process, however, is reversible. Removal of methyl-groups from cytosines in a promotor will allow the switch to gradually return to the ‘on’ position, or, if it had been switched off before, will allow a gene to be switched on (the gene will become activated). Again, how does this help in studying the ‘potential’? It would help, if a species experiencing a change in an environmental factor (e.g. a prolonged increase in ambient temperature) would respond by epigenetic activation (demethylation) of genes that improve the ability to cope with the new environment (e.g. higher temperature) and by deactivation (methylation) of genes that hinder such improvement. But it would help even more, if the epigentically ‘stored’ information an adult now has about the new environment (let’s call it ‘epigenetic memory’) could be transmitted to its offspring, thereby giving its offspring a better (well prepared) start into the new environment. After all, increasing the offspring’s chances of survival until the offspring starts reproducing is what Darwinian fitness is all about.
What should an experiment look like then? Take individuals of a species with a short generation time and let them reproduce to get baseline methylation patterns for the parents and the offspring. The patterns can be studied by comparing the sequences of a genome that had been treated with a chemical compound called bisulfite (which transforms all non-methylated cytosines into uracil, another building block) with a genome of the same individual that was not chemically treated. This way every single methylated cytosine can be detected. Then expose the parent individuals to a changed environment (e.g. increased ambient temperature or altered diet composition), and afterwards allow them to reproduce again (ideally in the same pairings as before). Now the methylation patterns are determined again. If we compare the patterns of the parents prior to, and after their exposure, we will learn about the epigenetic responses to the changed factor. If in different experiments different factors were altered, one can compare the different responses. But to learn if something like an ‘epigenetic memory’ exists, one would need to look at the methylation patterns in the offspring groups (one group born prior to the exposure, one born thereafter). Similar methylation patterns in the two offspring groups, of which neither one had been exposed to the changed environment itself, would clearly dismiss the concept of such ‘epigenetic memory’ and would also tell us that the ‘potential’ to cope with environmental changes is limited (as it then would depend on infrequent mutations only). The presence of different methylation patterns in the two offspring groups, however, would be a clear indication that the parents had conveyed (preparational) epigenetic information to their offspring.
We conducted such an experiment in wild guinea pigs (Cavia aperea). Because most research worldwide focuses on the transmission of epigenetic information from the mother to the embryo (e.g. maternal imprinting), we focused on the paternal (male) transmission. After their exposure to either an increased ambient temperature or a changed diet (both for 60 days) the fathers showed a clear shift in methylation patterns. The ‘temperature group’ activated genes from thermoregulation pathways, the ‘diet group’ activated genes in the energy synthesis and cell growths pathways. So the first question, if species can expand their genetic ‘potential’ to cope with environmental changes via epigenetic responses can be answered with a clear ‘yes’. In addition, we got an answer to a question we had not even asked. When we compared the responses to the two treatments (temperature increase, diet change), we found the factor-specific epigenetic response mentioned above, but in addition, we also identified genes that had been epigenetically modified in the same way in both treatment groups. Their modification was obviously independent of the environmental factor that we had changed experimentally. This reflects a more general response to changes in outer stimuli, independent of the type of factor that had changed. Comparing the two groups of offspring in each treatment and looking at the changes in methylation patterns showed the same picture as seen in the fathers, corroborating the idea of an ‘epigenetic memory’. Interestingly, although the fathers had prepared their offspring for a differing future environment, the changes in methylation patterns seen in the fathers (prior and after their exposure) were similar, but not identical to the changes in methylation patterns seen in the offspring (born prior to their fathers exposure or thereafter). This is good news for the ‘potential’ because it means that epigenetic responses are flexible and differ according to changes in the environment experienced by oneself, and that information is transmitted to the offspring to increase the ability of the next generation to cope with an already altered environment.
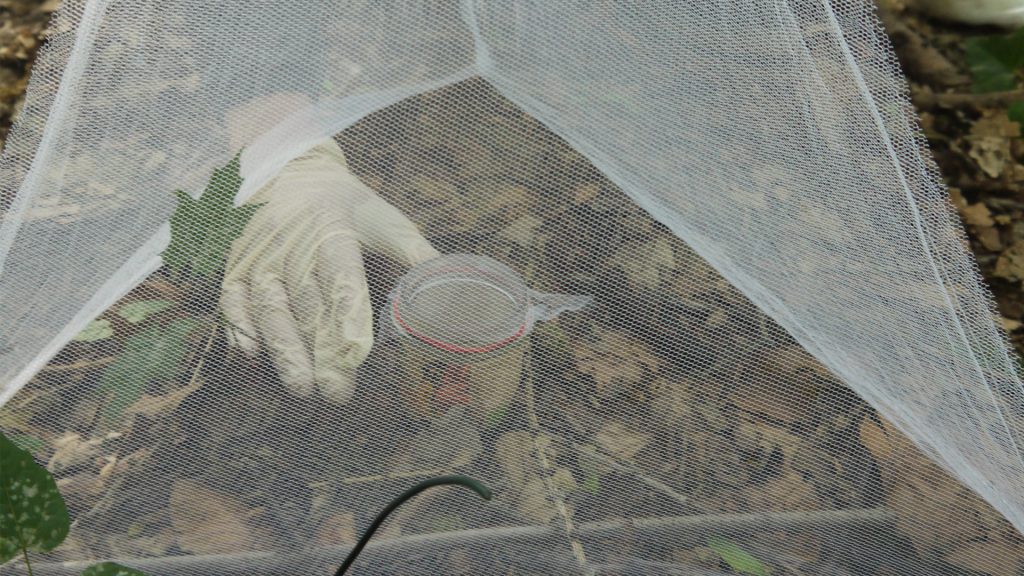
The importance of genomes
While describing the ways about how to assess the ‘potential’ of a species to cope with environmental changes, I have left out a very important topic. As we said in the beginning, the genepool of a species is the sum of the genetic information (genome) of all individuals. These individuals are very similar in their genomes, that’s why they belong to the same species. However, they also differ quite a lot in their genomes, as each individual has its own particular features (height, size, fur colour etc.). We have also already covered that mammals possess two copies of each gene (called alleles), one coming from one parent, the other coming from the other. To determine the genetic ‘potential’ of a species, it is not only necessary to determine which gene or group of gene codes for which trait, one also needs to determine the number of alleles that exist for these genes in the species. For example, in humans, there are three alleles for the gene ‘eye colour’ (brown, blue, green), while genes of the major histocompatibility complex have a much larger number of alleles, thankfully! This variability among the genomes of all the individuals of a given species is something that biologists call ‘genetic diversity’ of that species. This definitely needs to be taken into account when studying the ‘potential’, yet it is the hardest to achieve. It requires gaining access to genetic material from individuals of all populations a species consists of. However, access to remote areas may be very difficult, and the animals may be very elusive. In the latter case, alternative ways are required to gain access to genetic material. One is to collect DNA-containing material that was shed or dropped by the animals (hair, swabs of intestinal mucosal cells from faeces surfaces), another one is to employ haematophagous invertebrates (mosquitos, carrion flies, leeches) to obtain genetic material from host animals.
In an approach to determine the genetic diversity of the Eurasian lynx (Lynx lynx), Europe’s largest cat species, we were lucky enough to be able to rely on excellent collections and analyses already performed by colleagues from across Europe. We are interested in the Eurasian lynx, because it is a very charismatic (and unfortunately also very elusive) species that is returning to Europe via reintroductions and subsequent expansions from these core release areas. Because future environmental changes may negatively affect the success of the lynx to reclaim its former distribution areas, knowing the ‘potential’ for a sustainable recovery of lynx in Europe would clearly help to guide conservation measures (e.g. genetic composition of groups to be released). However, compiling all available genetic information regarding the different populations revealed that previous attempts to assess the genetic diversity of the Eurasian lynx had not covered all regions of the species’ distribution. Adding genetic information retrieved from hair and faecal swabs of Eurasian lynx living in regions not covered before to the analysis changed the phylogenetic tree quite a lot. In fact, the tree depicting the relationships among the populations (subspecies) and formerly consisting of two major branches (each branch consisting of populations of lynx that were closer related to each other that to any population of the other branch) ‘grew’ a new branch and one of the former two branches ‘grew’ quite a strong new twig. While we need to look into this much deeper now, what we already know is that the ‘potential’ of the Eurasian lynx is much larger than assumed previously.
For more information, please visit: www.izw-berlin.de/en/department-of-evolutionary-genetics.html
Please note, this article will also appear in the seventh edition of our quarterly publication.