International Editor Clifford Holt spoke to Oxford University’s Dr Katy Clough about how her STFC Ernest Rutherford Fellowship will enable her to investigate some of the biggest mysteries of the Universe.
The University of Oxford’s Dr Katy Clough is one of the recent recipients of the Science and Technology Facilities Council’s (STFC) Ernest Rutherford Fellowships, which are awarded to early career researchers who have leadership potential in their chosen field. The funding will enable Clough to investigate some of the biggest mysteries of the Universe, including the properties of dark matter, black holes and gravitational waves. She will also explore the behaviour of the Universe at the earliest times, when even small imperfections may blow up in a chaotic way. She will use supercomputing techniques to calculate how matter behaves in strong gravity environments to understand how we could have evolved from a messy Big Bang to the state of uniform expansion that we observe today.
The Innovation Platform’s International Editor, Clifford Holt, spoke to Clough about why this research is important and what she hopes to achieve.
Could you begin by explaining a little about numerical relativity and how you are working to provide numerical solutions to Einstein’s theory of general relativity? Why is it important to take this approach?
Numerical relativity is a set of computational tools which are used to solve the Einstein equation, which describes gravity as a curvature of the space and time in which we exist. The idea of solving gravitational problems on a computer sounds a lot like science fiction, with the computer being some kind of Skynet-like superintelligence that can understand things better than us mere humans.
Sadly, numerical relativity is not quite so futuristic – as most of us know from everyday experience, computers are (for the moment!) only as smart as the people who operate them. However, computers can do many simple calculations very quickly, and so by breaking a complex problem down into many small steps, a computer can work out a result that an army of humans would take years to compute.
Typically, the computer takes a certain initial scenario – two stars of a certain size, for example, moving towards each other – and works out how they will move over a very short period. In the Newtonian picture, a gravitational force accelerates them towards each other, so that they speed up a little. The end point of this step becomes the new initial scenario, and the calculation is repeated many times in a way that gradually tracks the motion of the objects. Each step could be done with a pen and paper but, especially where many such objects are involved, the overall result can give very complicated trajectories that could not simply be predicted at the start.
Einstein’s gravity does a similar thing, except that what moves is not just the astrophysical objects, but space and time itself – the motion of the stars creates waves that ripple off to infinity in all directions. These waves tell us a lot about the objects that created them, so we can learn about mysteries of the Universe by observing them. In particular, black holes can be detected in this way, and their properties measured.
It has only recently become possible to measure such gravitational waves on Earth – the Advanced LIGO/Virgo network of gravitational wave detectors made their first detection in 2015 – so this is a very exciting time to be working in the field. It is as if we have a new pair of glasses that allows us to see signals from space that were previously invisible to us. To really understand what we are seeing, we need predictions from numerical relativity simulations of how these signals should look for different events. Without such predictions, we cannot interpret what we see, and we cannot spot new physics that might tell us more about mysteries of the Universe.
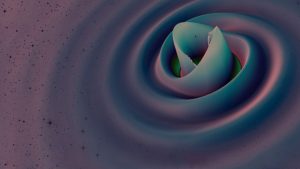
How do you plan to address some of the mysteries of the Universe, such as black holes and dark matter?
We know that the galaxy is partly made up a kind of matter that we cannot see with light, but that acts on objects gravitationally. We call this ‘dark matter’, which is a misnomer because as far as we can tell it is completely transparent to light, rather than absorbing it. Importantly, dark matter is not transparent to gravitational waves – or rather, having dark matter around when black holes merge will affect the gravitational signals that are emitted. The effect is very small, but such signals may turn out to be the only way to really understand whether dark matter is a particle, a wave-like fluid, or a collection of tiny black holes. By doing simulations of black holes in different dark matter environments, we can predict the impact on the gravitational wave signal, which can then be matched to observations to confirm or rule out the different theories of what dark matter could be.
As you are involved in the LISA consortium, how do you hope a space-based gravitational wave detector will help to answer some of your own research questions?
The best chance of seeing the impact of dark matter on gravitational waves is from its imprints on the signals of supermassive black holes, which exist at the centre of most galaxies including our own. The LISA space-based gravitational wave observatory targets the frequency of gravitational waves associated with these larger objects, and so if we are lucky, we may learn something about dark matter from its observations.
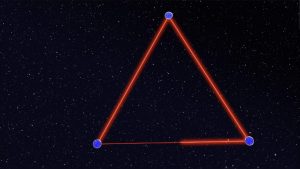
In particular, smaller black holes spiralling into supermassive black holes will experience a gravitational drag force from the presence of dark matter, and this has been shown to be detectable by LISA for certain wave-like models. Much more work needs to be done to investigate whether similar effects may arise in other competing theories, otherwise we will not be able to discriminate between them, and we risk mistaking their imprint for noise in the signal.
How do you plan to explore the behaviour of the Universe at the earliest times? What do you hope/expect to find?
Black holes are famous for containing ‘singularities’ – points where space and time end, which are surrounded by event horizons from which signals cannot escape. Another well-known singularity is the Big Bang singularity – if we naively rewind the history of our Universe in time, we reach a cosmological singularity where again we cannot continue any further. Roger Penrose’s singularity theorems tell us that the singularity cannot be avoided (subject to some caveats), but it does not tell us a lot about the nature of the singularity that we should encounter.
We can apply the same techniques used for studying black hole spacetimes to investigating the nature of this cosmological singularity. The focus of this aspect of my research is on understanding the caveats I mentioned. We may also discover that the singularity has features that naturally explain some of the mysteries of the Universe, like its tendency to be very homogeneous on large scales, or the fact that it is expanding at an accelerating rate.
How will the Rutherford Fellowship enable you to expand your research?
The areas I have described push the boundaries of gravitational research and leverage the development of new tools to solve long standing problems in fundamental physics. Having a five-year period of securely funded research allows me to develop these tools and take risks in finding creative solutions to the problems that will inevitably be encountered. It is also a starting point for leading a research group of my own, in a field that is going to be increasingly important internationally over the next decades.
What are your hopes for the future?
My biggest hope is that we see something completely unexpected in the gravitational wave data from future ground and space-based detectors! The fact that we can explain most of what has been observed with Einstein’s theory of gravity means that it is a good description of our Universe up to very high energy scales, which we had guessed but did not previously know for sure.
It is, of course, an amazing achievement to confirm what we expected to observe, and there have been a few surprises (such as the masses of the black holes observed). But for me, the most exciting thing would be to see a signal that really does not match any physical process we know. It would be a fun challenge to explain it.
What advice would you give to those who may wish to study similar areas?
The field of science belongs to everyone, and like art or literature, we can all learn to appreciate it at any age. Many people have learned science as a collection of dry facts – the names of planets, the distances to stars, and so on – and so they think it perhaps is not for them. Just like discovering that the Earth is not flat, but a pale blue dot in space, learning how our Universe is curved by black holes and evolves over time is not simply a dry fact – it is related with how we understand the connections between things, and how we see our place in the cosmos.
Because science is so beautiful and rewarding to study, I get annoyed when it is seen as something exclusive that is only for a certain type of person. I am constantly amazed by the insights that children and young people have when they ask questions freely, and without self-consciousness. I encourage everyone to hold on to their childhood curiosity about mysteries of the Universe and to seek out people who will generously and patiently share its wonders with you.
Dr Katy Clough
Postdoctoral Research Assistant in Theoretical Cosmology and Gravitational Physics
University of Oxford
01865 83017
katy.clough@physics.ox.ac.uk
Tweet @OxfordPhysics
https://www.physics.ox.ac.uk/our-people/clough
https://kaclough.github.io/
Please note, this article will also appear in the seventh edition of our quarterly publication.