Dr Anne Decourchelle of CEA Saclay outlines the intriguing science results from the CEA’s astrophysics department based on multiwavelength observations.
When it comes to the study of astrophysics, the first challenge is in accordance to gaining access to the full range of the electromagnetic domain. Ground-based observatories can be used in the radio and visible domains, as well as in few atmospheric windows in the infrared and submillimetre ranges. However, in the high-energy range (UV, X-rays and gamma rays) and in most of the infrared range, the Earth’s atmosphere blocks the electromagnetic radiation from the cosmos, requiring space observatories to observe the Universe.
The second challenge is the measurement accuracy needed in modern astronomy. This requires pushing the performance limits of the instruments, global detection system and data analysis, via innovative developments throughout the full detection chain, from instrument technologies to signal processing and control of systematics.
A brief voyage into some of the scientific issues in astrophysics will illustrate the strength and complementarity of a large wavelength range of observations, as well as multi-messenger observations.
From our Sun to exoplanet systems
Photons, produced during nuclear reactions in the Sun’s core, take millions of years to reach the photosphere, the visible ‘surface’ of the Sun, losing then all signatures of their initial conditions. To understand the Sun’s interior, it is necessary to use seismology (the study of wave propagation), which informs us about the properties of the cavities and the dynamics of the plasmas they pass through. The data from space missions (such as SoHO, SDO or CoRoT and Kepler/K2) improve our knowledge of the physical processes that control the evolution of stars and provide constraints to theoretical models.
Stars are dynamic, turbulent and magnetic objects. Understanding the origin of this magnetism is essential because this intense activity, via the stellar wind and magnetic eruptions, has a direct impact on the environment around the stars. The magnetism of the Sun sculpts its environment. A rapid wind of particles escapes from coronal holes, accelerating away from the Sun up to speeds of 800 km/s. How this wind is accelerated is one of the fundamental questions of solar physics that the recently launched NASA’s Parker Solar Probe and ESA’s Solar Orbiter (ESA) missions are seeking to elucidate with a large set of complementary instruments.
A large majority of stars has exoplanets orbiting around them. Knowing the host star allows to better understand its planet(s) and habitability conditions. The future looks bright thanks to NASA’s TESS space mission, launched in 2018, ESA’s Cheops mission in 2019 and the preparation of ESA’s future mission, PLATO, which will observe millions of stars, including dozens of stellar systems like ours. In addition, the exoplanets characterisation will make a giant leap with the NASA’s JWST to be launched in 2021 and the ESA’s ARIEL mission in 2028.
From the interstellar medium to the formation of stars
Star formation is complex. It involves gravity, turbulence and magnetisation of the interstellar medium, feedback phenomena and the thermodynamics of interstellar gas. It occurs within the densest interstellar gas and dust clouds in galaxies. By probing these clouds in the far-infrared and sub-millimetre range, new links between the mechanisms of star formation and those structuring the interstellar medium at large scales have been discovered. The quasi-systematic presence of disks of matter around protostars, disks where exoplanets are formed, have also been observed.
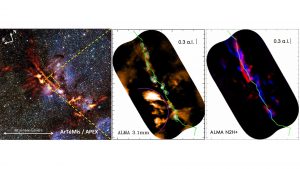
ESA’s Herschel satellite have revealed a profusion of interstellar filaments as well as numerous dense ‘pre-stellar’ cores along these filaments. With complementary ground-based observations (IRAM, APEX/Artemis, ALMA) shown in Fig. 1, and theoretical models, a two-step scenario for solar-type star formation has been constructed. In the first stage, a series of multiple compressions associated with large-scale supersonic ‘turbulent’ motions generates a complex entanglement of filaments in each interstellar cloud. In a second step, gravity takes over and fragments the densest filaments into pre-stellar cores, which transform into protostars and then into stars like the Sun.
Although many details remain poorly understood, this scenario provides a very serious path to explain the origin of the characteristic mass of stars and the remarkably uniform efficiency of star formation in the dense molecular gas of galaxies. It postulates that the characteristic mass of stars would be a direct result of the characteristic mass of filament fragmentation and that the ‘microphysics’ of the fragmentation process would play a ‘universal’ role in regulating the efficiency of star formation in the dense molecular gas of galaxies such as the Milky Way.
From exploding stars to their supernova remnants
High energy astrophysics allows the study of the most violent phenomena in our Universe: star explosions and formation of compact stars (pulsars, magnetars, black holes), shocks and acceleration of cosmic rays, accretion and relativistic ejections by compact stars. These objects are laboratories of extreme physics.
By looking at the traces left in X-rays by star explosions, we decipher from the supernovae remnants the conditions of the explosion, the nucleosynthesis of heavy elements, the hydrodynamic instabilities generated by the explosion, and their impact on the surrounding environment. The largely supersonic shocks, generated by the ejection of material, accelerate particles, and are probably one of the main sources of galactic cosmic rays. These particles produce emission in the gamma-ray domain, respectively leptonic for the accelerated electrons and hadronic for the accelerated ions. Joint studies of high (GeV) and very high (TeV) energy gamma-ray emission, with NASA’s Fermi mission and HESS ground-based Cherenkov telescope respectively, have shown two main categories of supernova remnants emitting gamma rays.
On the one hand, often young (less than 5000 years old) remnants have a gamma-ray spectrum dominated by a lepton emission and a shock wave evolving in a low-density ambient environment (~0.1 cm-3). On the other hand, there are supernova remnants interacting with a denser interstellar medium (more than 1 cm-3) and whose gamma ray spectrum is dominated by a hadronic process. This shows that both the age and the environment in which the SNR evolves plays as important a role on its high-energy gamma-ray emission.
From clusters of galaxies to cosmology
Cosmology aims to develop a scenario that allows us to trace the origins of the structures in the Universe. It uses the Universe as a laboratory where we can test the fundamental laws of physics as well as the existence of components that are still undetectable in the laboratory, such as dark matter and dark energy.
A simple view of structure formation model relies on the fact that when the density of matter in a given region of the Universe becomes sufficiently high, it dissociates from the expansion and begins to undergo a gravitational collapse. This hierarchical process leads to the formation of stars, galaxies, groups of galaxies and, finally, clusters of galaxies (which appeared when the Universe was only about a quarter of its present age).
Galaxy clusters play a key role in cosmology. They contain gas and dark matter which can be characterised via X-ray observations. In a cluster at equilibrium, the gravitational force due to dark matter is compensated by the gas pressure. Images obtained by ESA’s XMM-Newton and NASA’s Chandra space observatories provide accurate maps of the gas density distribution, while the X-ray spectrum determines its temperature (several tens of million degrees). From these, precise measurements of the distribution of dark matter in clusters can be obtained up to about eight billion years ago. These dark matter profiles have a peaked density at the centre, as predicted by cosmological numerical simulations, and are surprisingly similar to those observed in objects at the present time.
I presented a few examples of the need to observe in several wavelengths and to push the instrumental limits to understand the Universe. The recent detection of gravitational waves is an illustration of it, opening a new era of multi-messenger observations, and will undoubtedly bring new key complementary measurements in astrophysics.
Anne Decourchelle
Director
Department of Astrophysics/AIM,
CEA Saclay
+33169084384
anne.decourchelle@cea.fr
http://irfu.cea.fr/dap/
Please note, this article will also appear in the first edition of our quarterly publication, the Innovation Platform.