Adam Welch, Orbital Analyst at Raytheon NORSS, discusses the challenges of ensuring Space Situational Awareness and outlines how NORSS’ sensor technologies can assist.
Currently, there are 27,504 publicly monitored Resident Space Objects (RSOs), including active satellites, decaying satellites, space debris, and more. This does not include many classified satellites not publicly acknowledged. From 30 May 2023 to 30 June 2023, an estimated 22,723 conjunctions, i.e., close approaches between two orbiting objects, occurred.1 Both these numbers are increasing year on year, with the risk of a Kessler Syndrome2 event rising ever higher. Compound this with the increasing capability and number of Anti-Satellite (ASAT) capable nations3 and a noted increase in the militarisation of space,4 and it becomes apparent that the space environment is becoming more hazardous daily. In the best case, the risk to satellite failure is increased; in the worst case, the space debris becomes so prominent that space operations become practically unfeasible. Space Situational Awareness (SSA) is the means to maintain awareness of the space environment, and to understand and characterise the risks posed to spacecraft and space operations.
What is Space Situational Awareness?
SSA aims to monitor, track, and predict RSO positions, movements, and status. Understanding where satellites are, their status, and where they are going enables space sustainability to be improved by minimising collision risks, modelling/predicting satellite re-entries, and mitigating the effects of space debris. Further expanding upon SSA leads to monitoring the ownership, actions, and intent of military satellites. This is often referred to as Space Domain Awareness (SDA).
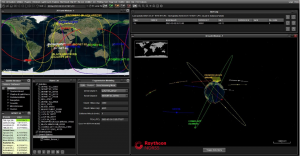
Space Situational Awareness is a growing field of interest to both government and private entities across the globe, with numerous pieces of hardware and software designed to aid in SSA activities and reporting. Government agencies aim to understand the risks posed to national space assets, ranging from asset damage to operational security of space systems, whilst private agencies seek to protect commercial assets and potentially sell SSA data to other private agencies or government organisations. One of the primary goals, however, for most parties is the safety and security of their space assets whilst ensuring the space environment remains sustainable for future use. Numerous hardware and software solutions enable effective SSA.
Raytheon NORSS is at the forefront of end-to-end SSA, defined here as performing data acquisition through sensor operations, data processing, and data analysis. Raytheon NORSS has designed and deployed a bespoke optical SST sensor system, namely LOCI, enabling the acquisition of data as a significantly cheaper alternative to many typically expensive, limited access, sensor systems and/or purchasable datasets of limited availability. Data processing is then performed in-house, converting the raw astrometric data acquired into valuable processed SSA data that can be input into analytical tools and software.
Raytheon NORSS possesses numerous bespoke analytical software tool suites – the most prominent being the orbital analysis tool suite, NORSSTrack. NORSSTrack is a bespoke astrodynamics software designed in-house to model, critique, and understand RSOs, their current and future movements, and any related events of interest. These tools are complemented by skilled orbital analysts who perform analysis to identify the relevant characteristics, properties, and outputs desired to increase our understanding of the operational space environment. The NORSSTrack tool suite offers various products and capabilities, including space-data visualisation; conjunction analyses; re-entry analyses; fragmentation and breakup analyses; satellite manoeuvre detection; catalogue compliance monitoring; sensor modelling and simulation, as well as many more SSA analytical capabilities.
Raytheon NORSS Space Situational Awareness software and services can also aid future spacecraft operations by modelling orbital lifetime analysis, collision avoidance plans and disposal options based on parameters provided, via in-house risk analysis software, NRAD. Additionally, SSA sensor design and deployment is supported by means of comprehensive ground- and space-based sensor surveys with the bespoke BALDR simulation suite. The above capabilities represent a handful of analytical solutions that can be provided by Raytheon NORSS and its diverse SSA portfolio. Examples of the capabilities of Raytheon NORSS tools are presented below:
Sensors in space
Critical to Space Situational Awareness is the use of effective sensors. Numerous sensor technologies exist, each designed with different requirements, targets, mission objectives, and operational methodologies in mind.
Typically, sensors can be divided into two groups: ‘passive’ or ‘active’. Passive sensors measure naturally-occurring emissions, reflections, and transmissions from an object (such as light from the Sun reflecting off a satellite), whilst active sensors send out energy and measure the changes in the energy that returns (such as with active radar systems e.g. Synthetic Aperture Radar). Depending on the information desired, different sensor technologies and techniques should be employed. For example, analysing the streak of light produced from a moving RSO (called Light Curve Analysis) can determine the RSO’s attitude stability, whilst close-proximity, detailed images (called Resolved Imaging) can help identify the physical state of a satellite. Continuous observation of a specified region of space (called Wide-Field Surveys) may help identify the movements, and potential conjunctions, of satellites whilst observing a satellite across multiple spectrums of light (called Multispectral Imaging or Hyperspectral Imaging) can help identify material properties of a satellite. Different objectives thus mandate different types of sensors.
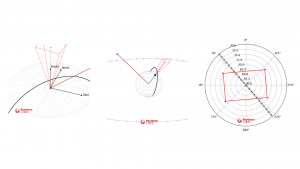
When considering ground-based sensors, the most common sensor types, however, are typically radar or optical sensors. Radar is a powerful sensor type, typically used in detecting Low Earth Orbit (LEO) targets. Radar sensors are largely unaffected by lighting and weather conditions, can determine distance to a target, and are capable of tracking multiple objects simultaneously.5 Radar constitutes most ground-based sensor sites utilised in the US’ Space Surveillance Network (SSN). The core issue with radar sensors, however, is the significant power requirement. Optical sensors are typically, though not exclusively, used to observe Geostationary Earth Orbit (GEO) targets. Optical sensors are significantly cheaper than radar, allow for tracking of high-altitude satellites, and do not demand the vast power requirements to observe GEO targets that radar systems would demand due to the immense distances involved. Optical telescopes, however, are limited in operational capacity based on both lighting conditions and weather conditions. Other sensor solutions that exist, such as laser or infrared sensors, are typically less developed, more expensive, and/or more unique in their use-case than their optical and radar counterparts.
Ground-based sensors, however, are limited in their capabilities due to numerous factors. From the weather and lighting condition requirements, to scattering and attenuation of signals due to Earth’s atmosphere, to an inability to place a sensor site in an optimal location due to geographic or political constraints. These are merely a few of the issues that arise when utilising ground sensors. Space-Based Space Situational Awareness (SB-SSA) sensors circumvent many of these issues, with capabilities such as an increased revisit rate (i.e. how frequently a target is observed), the ability to see dimmer and smaller objects due to the lack of atmospheric interference, a significantly increased number of observations than ground-based counterparts due to the lack of weather effects and a longer window for targets to remain illuminated by the Sun. Furthermore, the technology is proven, as many missions such as Sapphire, SBSS, GSSAP, and more have all utilised SB-SSA sensors effectively.6
SB-SSA sensors, much like their ground sensor counterparts, typically consist of optical and radar sensors, however, their implementation differs drastically given the space environment. Whilst in principle SB-SSA sensors are highly effective, there are numerous challenges that must be understood and considered when employing these technologies in an operational space environment. These challenges can be broken down into three general categories: social, sensor, and spacecraft challenges.
Social challenges to SB-SSA
Firstly, cost is a significant factor. The design, construction, launch, operation, and eventual disposal of a single spacecraft is a lengthy and costly process, typically far exceeding the costs behind implementing a single ground-based sensor. Should an SB-SSA sensor network utilise a satellite constellation, which could drastically enhance performance, costs spiral even higher.
Finance notwithstanding, the significant development time cost must also be accounted for. Starting with a mission plan and proceeding from pre-development designs to the eventual launch of a satellite is a lengthy endeavour, with typical industry timelines taking roughly two to three years. One must be certain that the value of the data acquired is worth the long wait. Additionally, despite the long development time, it must be recognised that satellites are temporary and inevitably cease functioning. Satellites at their end of life, functional or not, must either decay or be placed in a graveyard orbit. Due to the increasing number of satellites operating within Earth’s orbit, operators are heavily encouraged to appropriately dispose of their satellite as soon as all valuable data has been obtained. Mission planners must ensure that all mission objectives are met in a timely manner. Certain spacecraft, such as the MEV, can extend a satellite’s lifetime significantly, however such continued servicing is extremely expensive and not always permitted, thus missions cannot be designed with this in mind.
Sensor specific challenges to SB-SSA
As highlighted previously, radar systems demand high power draws; ground-based radars can draw average powers into the 100s of kW, whilst spacecraft typically need a few kWs at most. Consequently, spacecraft are often unable to fully utilise their radar systems and require time to recharge batteries as part of their duty cycle, thus preventing spacecraft radar systems from reaching their potential.
More dramatically, both optical and radar sensors risk damage in space due to debris striking the exposed sensor. A pertinent example is that of the Copernicus Sentinel-1A satellite which suffered damage 40cm in diameter to its solar panels due to a 1mm debris particle impact.7 Whilst not directly striking the sensor, this example notes that damage to other components can also render sensors hindered or inoperable due to the space environment. Even solar flux, after significant exposure, can begin to damage optical lenses; the space environment possesses significant risks not present when operating ground-based SSA sensors. Additionally, ground-based sensors can easily be adapted and modified as needs demand, whilst SB-SSA sensors must be selected and designed for a precise, largely inflexible function. A particular example is in the choice of aperture size. A large aperture size allows more light to be collected by an optical sensor, thus identifying dimmer objects, and requiring a lower exposure time. This, however, may be problematic if the exposure time is too long due to mission/spacecraft/payload constraints or if the payload mass or volume budget is small, as large apertures typically require large lenses to let in the requisite light.
Spacecraft specific challenges to SB-SSA
Naturally, one of the defining limitations of a SB-SSA payload is the size, weight, and power (or SWaP) available to it. Spacecraft allocate SWaP values for each subsystem including the payload subsystem. The SB-SSA sensor itself is only a part of that subsystem with an even smaller SWaP allocated to it.
Thus, one must select or design their spacecraft carefully to avoid being overly constrained by said spacecraft (or vice versa in designing the SB-SSA sensor).
Critically, however, will be the storing and downlinking of SB-SSA data obtained by the sensor, which will be primarily dependent on the amount of data acquired over a given operational timespan. In the short term, enough data storage must be available to hold the requisite data before downlink, an issue that would naturally not be present for ground-based sensors. Depending on the amount of data acquired, a minimum revisit rate may also be required to ensure enough time is available for data to downlink from the satellite to the ground stations. The choice of transmission band will naturally affect this, as bandwidth determines the data downlink rate. Higher frequency transmission bands, such as the X, K, and Ka bands, do not inherently have larger downlink rates, however they typically have more bandwidth available around the selected frequency, meaning they tend to have higher downlink rates.
SB-SSA: Final thoughts
It must be noted that these challenges do not render SB-SSA sensors ineffective, inefficient, or unvaluable. The challenges presented are merely the focus points for future SB-SSA sensor development. The vast increase in the number of possible observations, the rapid revisit rate of observing targets, and the mitigation of atmospheric, weather, and lighting effects encountered by ground-based sensors highlight the great value presented by SB-SSA sensor solutions. Furthermore, by developing and investing in SB-SSA sensors, new technologies and techniques are likely to emerge which could drastically expand upon SSA capabilities for both ground-based and space-based systems. Innovative missions with unique objectives, especially in the military domain, necessitate the deployment and operation of SB-SSA sensors.
Given the significant advantages presented by SB-SSA sensors, the future of SSA and SDA becomes more clear. As these challenges are overcome, a likely new era of space sustainability will emerge, with SB-SSA at the forefront of understanding and protecting Earth’s space environment and the invaluable resource space provides to modern society today.
References
- Space-Track, “The Satellite Situation Report” (Accessed: 04/07/2023)
- Wall M., “Kessler Syndrome and the space debris problem” (Accessed: 04/07/2023)
- Secure World Foundation, 2021, “Anti-Satellite Weapons Threatening The Sustainability of Space Activities” (Accessed: 04/07/2023)
- Mills C. and Butchard P., 2021, “The militarisation of space”, UK Parliament, UK
- Secure World Foundation, 2022, “Space Situational Awareness – A Quick Overview” (Accessed: 04/07/2023)
- 18th Space Control Squadron, 2019, “Space Surveillance Network” (Accessed: 04/07/2023)
- European Space Agency, 2016, “Copernicus Sentinel-1A satellite hit by space particle” (Accessed: 04/07/2023)
Please note, this article will also appear in the fifteenth edition of our quarterly publication.