Professor Pedro Schwaller and Wolfram Ratzinger from the Institute of Physics at Johannes Gutenberg-Universität Mainz, discuss the recent detection of very low-frequency gravitational waves
The North American Nanohertz Observatory for Gravitational Waves (NANOGrav) collaboration uses pulsars – exotic, dead stars that send out pulses of radio waves with extraordinary regularity – to detect gravitational waves. In a recent analysis of data obtained by the collaboration, which appeared to contain the first signs of very low-frequency gravitational waves, Professor Pedro Schwaller and Wolfram Ratzinger from the Institute of Physics at Johannes Gutenberg-Universität Mainz, have considered the possibility of whether this may point towards new physics beyond the Standard Model.
The Innovation Platform’s International Editor, Clifford Holt, spoke to the physicists about the importance of detecting gravitational waves at these frequencies, as well as the impact of the signal potentially being consistent with both a phase transition in the early Universe and the presence of a field of extremely light axion-like particles (ALPs), the latter of which are considered as promising candidates for dark matter.
Can you tell me a little about NANOGrav and why the detection of very low frequency gravitational waves (GWs) is important?
Just like light waves, gravitational waves of different wavelengths allow us to investigate different phenomena/sources. When thinking about astrophysical sources of GWs like inspirals of massive objects, the importance is easy to understand: bigger and therefore heavier objects emit GWs with bigger wavelengths and lower frequency. While the inspirals we observe at LIGO involve objects with only several solar masses, NANOGrav can potentially look for inspirals of black holes at the centre of galaxies weighing billions of solar masses. For the primordial sources, that is sources in the plasma that filled the early Universe that we are interested in, the relation is not so apparent, but processes that happen later when the temperature of the plasma is lower give lower frequency GWs. Therefore, by searching for gravitational waves across a broad range of frequencies, we can probe different epochs of the early Universe shortly after the Big Bang.
The NANOGrav collaboration has detected, for the first time, gravitational waves at the very low frequency of 10^-8 Hertz. Why is this important? What can you learn from this?
GWs only interact very weakly. They are therefore hard to source and detect. Now that the detection has been accomplished, we know that there has to be a source for GWs of this wavelength. As the data gets better, we will learn more about the features of these gravitational waves and therefore the nature of the source. We therefore expect to gain insight into astrophysics as well as cosmology. The particular frequency that NANOGrav is sensitive to corresponds to a specific time in the early Universe when the interactions become strong (i.e. quarks and gluons start to form protons, neutrons, and nuclei). If the signal is confirmed, it would point to other, so far unknown, processes that take place at the same time and that could, for example, be responsible for the production of dark matter.
What were the biggest challenges involved in making this detection?
This detection relies on the ability to consistently monitor the arrival of light pulses from rotating stars that act like a lighthouse, so-called ‘pulsars’. To find a sufficient number of appropriate stars, a state-of-the-art radio telescope or even an array of them is needed. As for the consistency of the data taking, there is really not much one can do but wait. The sensitivity of pulsar timing arrays gets better as one goes to lower frequencies. The frequencies of the gravitational waves that were detected correspond to periods of approximately ten years, which is why data from more than one decade was needed. There has also recently been progress in the understanding of so-called ‘noises’ in the data taking process, which could mimic a signal. This understanding is key in claiming a detection.
Phase transitions in the early Universe and a dark matter field of extremely light axion-like particles (ALPs) are thought to account for two possible scenarios that could have caused the observed GWs. Can you tell me a little about each – and perhaps why/if you feel the latter is more plausible?
Phase transitions are an everyday phenomenon: the melting of ice and the boiling of water, if we add enough energy (in the form of heat), are examples of phase transitions. The transition from a gaseous to a solid phase is associated with the breaking of a symmetry since the crystalline structure of a solid has a preferred direction.
Our description of Nature at the most fundamental level relies on symmetries. While some of those symmetries are broken today (for example the electroweak symmetry that underlies the electroweak interactions), we know that these symmetries were unbroken in the early Universe at very high temperatures. Several such symmetry breaking phase transitions happened in the early Universe. If these transitions are of first order, i.e. particularly violent, they produce a stochastic gravitational wave signal, similar to the one observed by NANOGrav. Since none of the phase transitions in the Standard Model of particle physics are of the first order, this would be an indication of new physics. In addition, these phase transitions could explain why today there is no anti-matter in the visible Universe.
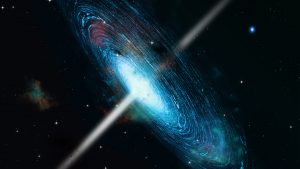
Axion-like particles are very light particles that only interact feebly with the known particles in the Universe. Their existence is predicted in many fundamental theories of Nature, such as in string theory. Via the so-called ‘mis-alignment mechanism’, they can be sufficiently abundant today to explain all of dark matter. We have recently shown that in some axion models the dynamics of the mis-alignment mechanism also leads to the production of potentially detectable gravitational waves. The most probable mass range in fact is consistent with the frequency of gravitational waves that was now observed by NANOGrav. This was one of the main motivations to immediately sit down and find out whether the signal shape would be consistent with our predictions – which we found to be the case. At this point, we think it is too early to say whether one or the other scenario is favoured by the data.
Given that gravitational waves are being held up as a new frontier in astronomy, what are your hopes for the future of gravitational wave astronomy – and, indeed, for NANOGrav?
All of what we know about the early Universe so far comes from observations of electromagnetic radiation – i.e. light. And with that tool we can only look back until 300,000 years after the Big Bang, anything that happened earlier can only be inferred indirectly or is based on purely theoretical calculations. Gravitational waves could be a game-changer here: since gravity is so weakly coupled, any gravitational waves emitted even mere seconds after the Big Bang will persist today and could eventually be observed by our GW detectors. This could potentially revolutionise our picture of the very early Universe and maybe even provide some guidance about possible theories of quantum gravity.
For NANOGrav, it would of course be great if they can confirm their discovery with more data. This would open the nano-Hertz frequency band for GW astronomy. Currently, we are only sensitive to frequencies in the LIGO band, around 100Hz, which very much limits the types of gravitational wave sources we can observe. In comparison, for electromagnetic waves, we constantly observe the sky at a broad range of frequencies from radio-waves over the visible light all the way to X-rays and gamma-rays. So, adding a second frequency band will be like opening a second pair of eyes!
Please note, this article will also appear in the sixth edition of our quarterly publication.